
An official website of the United States government
The .gov means it’s official. Federal government websites often end in .gov or .mil. Before sharing sensitive information, make sure you’re on a federal government site.
The site is secure. The https:// ensures that you are connecting to the official website and that any information you provide is encrypted and transmitted securely.
- Publications
- Account settings
Preview improvements coming to the PMC website in October 2024. Learn More or Try it out now .
- Advanced Search
- Journal List
- Springer Nature - PMC COVID-19 Collection


Indian Sugar Industry: Towards Self-reliance for Sustainability
ICAR-Indian Institute of Sugarcane Research, P.O. Dilkusha, Lucknow, Uttar Pradesh 226 002 India
The South-Asian region including India is a major hub of sugar producing countries with ample presence in the global sugar scenario. India has a rich history of sugarcane and sugar production since time immemorial, and the industry has gradually evolved to find a place among the top sugar producing countries of the world. The innovative technological interventions for sugarcane improvement, production and management have helped the industry to progress towards a diversified and bio-based productive, sustainable and profitable one, thereby gradually becoming self-reliant. This self-reliant industry with the right mix of linkages and collaborations, has been successful in tackling the various unforeseen challenges including those that cropped up during COVID-19 pandemic. The industry also fulfils its Corporate Social Responsibilities leading to the overall betterment of its stakeholders. This has enabled the Indian sugar industry to align itself with the 2030 Agenda for Sustainable Development Goals.
Introduction
India is a major sugar producing country in the South-Asian region and has recorded bumper sugar production in the recent past. It has a strong sugar sector backed by a well-developed R&D set-up. This has helped the industry overcome most of the challenges, be it in the area of production, processing or other related sectors. Even from very early period, as mentioned in medieval literature, India has been cultivating sugarcane and processing the cane. Slowly innovative technologies were developed to suit the time-based and location-specific demands that facilitated India to become a major player at the global level. The sugar industry is inherently inclusive with the crop occupying around 5.0 million hectares, i.e. 2.57% of the gross cropped area and supporting over 7 million farmers and their families, along with workers and entrepreneurs of over 550 sugar mills. In India, sugar is an essential item of mass consumption, and the domestic demand is around 27 million tons per annum. Sugar and jaggery are the cheapest source of energy, supplying around 10% of the daily calorie intake. Sugarcane has been projected as the crop for the future contributing to the production of not only sugar but also as a renewable source of green energy in the form of bio-fuels, bio-electricity and many bio-based products. The industry produces 370–400 million tons (MT) cane, 27–30 MT white sugar and 6–8 MT jaggery and khandsari every year. Besides, about 3.2 billion litres of alcohol and 4700 MW of power and many chemicals are also produced. The industry has the capability to export around 3500 MW of power to the national grid. Sugar industry has gradually transformed into sugar complexes by producing sugar, bioelectricity, bioethanol, biomanure, bio-CNG and chemicals. The Indian sugar industry has been capable of meeting the sweetener and energy demand of the nation and to a great extent, has become self-reliant, showing the path to the other countries in building a productive, profitable and sustainable industry (Solomon 2014 ).
Indian Sugar Industry: The Pre-independence Era
Sugarcane was grown in India from the Vedic period even though there are no reports of a well-organized sugar industry at that time. Atharva Veda (1500–800 BC) has numerous references about sugarcane in India, while the famous historians like Herodotus mentioned about the crop in their works. Ibn Battuta and Francois Bernier were other famous voyagers who wrote about the sugarcane cultivation in Kerala and Bengal. Tamil literature also has several references about sugarcane. The technique of boiling sugarcane juice to extract sugar was first discovered in India during the first millennium BC. Mention of establishment of first sugar factories in India can be found as early as 1610 at Masulipatnam and Coromandel coast and subsequently at Surat in 1912. There are unconfirmed reports of the initiation of the first sugar plant in Orissa in 1824 by the French. In 1904, the first modern sugar processing unit was established at Saran in Bihar, with the setting up of the first vacuum-pan process plant (Mishra 2018 ). The performance of the industry during the period demanded much improvement. With the setting up of Tariff board in 1931–1932, the colonial government imposed 185% ad valorem import duty on the sugar imported from Java and this was followed by a substantial increase in the number of sugar factories in the country. The number of factories rose from 29 to 137 between 1931 and 32 and 1936–37. There was an increase of more than 300% in their numbers, with around 140 factories in 1946–47. A corresponding increase in the sugar production was also realized, from 1.61 lakh tons to 9.5 lakh tons during this period. The sugar production recorded a CAGR of 11.62% during the period. The average capacity of the mills increased at a compound rate of 1.61% with a corresponding increase of 0.75% for the sugar recovery. There was a substantial increase in the other factors like sugarcane acreage (CAGR 0.67%), cane production (1.01%) and cane yield (1.09%) (Reviewed in Mishra 2018 ).
Post-independence Era
The post-independence era also witnessed remarkable developments with respect to the sugar sector. The R&D sector scaled new heights with new research organizations being established. Special programmes were initiated to overcome the upcoming challenges like disease and pest incidence in this crop. Development of improved sugarcane varieties with desirable traits, application of novel agro-techniques for crop production and management, mechanization of sugarcane agriculture, modernization and improvement of performance of the sugar mills, etc., contributed to an overall improvement in the cane production and productivity. By the end of last century, the sugarcane area in the country increased to 4.4 million ha, with the cane yield touching 68 t/ha. The sugar production in 2000 was 18.2 million tons. Further developments in terms of varieties and technologies including ICT-based technologies in the sugar sector led to a quantum jump in sugar production, with a record production of 33 million tons in 2018–19 (Anon. 2021 ). Promising varieties like Co 0238 that have resulted in an improvement of 102% sugar recovery during the period from 2012 to 2019–2020 (Ram and Hemaprabha 2020 ) are an example of how the technological advancement in the sugar sector has revolutionised the industry. The area under the crop increased over 300% (1.71 million ha in 1950–51 to 4.841 in 2019–20. The yield of sugarcane during this period has gone up from 40.5 tons/ha (1950–51) to 71.1 t/ha in 2019–20. The sugar recovery has gone up by around 10% from that during the ‘50 s and ‘60 s. Presently, many innovative, sustainable and promising technological interventions like better planting techniques, integrated resource management strategies, bio-intensive plant protection methods, information technology-driven user-friendly computer/mobile phone-based applications for precision agriculture, mechanization, etc., are being put to use in the sugarcane cultivation scenario. A high level of modernization has taken place in the processing arena, enabling the sugar processing units to perform better, at the same time delving into product diversification. Transition towards a “Green Smart Sugar-Agro Complex” with an emphasis to produce green energy and other bio-based products apart from sugar, is the hallmark of the present-day sugar factories. All these had been possible due to the constant efforts of the sugar sector to evolve and excel and to become self-reliant to the maximum extent possible. The constraints faced by the industry during the COVID-19 pandemic (Solomon et al. 2020 ) have also been an important reason for the Indian sugar industry to move towards self-reliance.
The sugar industry has also been involved in the betterment of life in the sugar mill command areas. Most of the sugar factories are generally situated in rural settings, with limited basic facilities being available in the sugar mill operational areas. This was perceived by the sugar industry as a golden opportunity to take up developmental activities in these areas, ensuring the socio-economic development of the industry beneficiaries in their respective mill operational areas. As a part of their professional commitment, the mills promote a better living for its beneficiaries through their many developmental programmes. The mills also conform to the National Green Tribunal (NGT) standards, thereby facilitating a healthy clean environment in the area. The rural industrialization brought about by diversification and other activities has also enhanced employment opportunities. This also has brought in an element of self-reliance in the society. Thus, sugar and related industries have been important components of the social fabric in the pre-and post-independence era, contributing towards the country’s economy. The sector has been instrumental in social development, mobilization of resources and employment generation and has been from time immemorial, a significant player in fulfilling the Corporate Social Responsibility (Solomon et al. 2019 ) and integration of the Sustainable Development Goals of Agenda 2030. This is true not only in India, but also in many of the sugarcane growing countries all over the world, especially those in South Asia, that are major players in the global sugar markets.
R&D Network in Sugar Sector
India has a very strong R&D network comprising the national sugarcane research institutions (ICAR-Indian Institute of Sugarcane Research at Lucknow, Uttar Pradesh, in the sub-tropical region and ICAR-Sugarcane Breeding Institute at Coimbatore, Tamil Nadu in the tropical region), research centres funded by state government, research institutes by private organizations and sugar mills, State Agricultural Universities and their research centres, etc. Apart from these, the All India Co-ordinated Research Project (AICRP) on Sugarcane co-ordinates the sugarcane research activities through its network of centres in the different states all over the country. Through its network of 22 regular and 15 voluntary centres, AICRP on Sugarcane aims at pooling the research resources of the country involving SAUs, Central and State Sugarcane Research Stations as well as Non-Government Organizations in a national grid for addressing the problems of regional and national importance. This entire R&D network forms the major source of a well-trained and highly efficient human resource, thereby facilitating the innovative and cutting edge research in the areas of sugarcane improvement, production and management, including the diversification (Sinha 2016 ) . These research institutions are well equipped to cater not only to the national demands for capacity building, but also for the international stakeholders. In addition, there are institutions like National Sugar Institute (NSI), Kanpur, and Vasantdada Sugar Institute (VSI), Pune, that impart quality technical education and also act as a rostrum to develop human potential to its greatest degree in the field of sugar processing, alcohol technology and diversification.
Technological Interventions for Self-reliance in Indian Sugar Industry
Development of improved sugarcane varieties.
Sugarcane varietal development is a very significant component of the sugarcane R&D in India. The nation has several research organizations, viz. ICAR-Sugarcane Breeding Institute (ICAR-SBI), Coimbatore, considered as the cradle of sugarcane breeding, ICAR-Indian Institute of Sugarcane Research (ICAR-IISR), Lucknow, which caters to the sub-tropical part of the nation, Vasantdada Sugar Institute (VSI), Pune, state funded research institutions like Uttar Pradesh Council of Sugarcane Research (UPCSR), Shahjahanpur in Uttar Pradesh, the various sugarcane research stations under the State Agricultural Universities (SAUs), etc., that take care of the location-specific as well as the nation-wide demands.
In India, through a well-organized network of All India Co-ordinated Research Project (AICRP) on Sugarcane, around 116 improved sugarcane varieties have been identified and approximately 60 varieties have been notified for commercial cultivation from 2000 till now. All these have been developed by the researchers in India, and many of these varieties are under cultivation in large areas, for example, Co 86032 (70%) in Peninsular Zone, Co 0238 (55%) in North West and North Central Zones, CoLk 94184 (28.25%) in North Central Zone, CoM 0265 (18%) in Karnataka, CoOr 03151 (18%) in Odisha & Co 06030 (18%) in East Coast Zone (Shukla et al. 2018 ). The recently notified varieties like CoLk 11203, CoLk 12207, CoLk 12209, CoLk 14201, Co 11015, etc., have begun to show their worth in the respective sugarcane growing zones. This speaks volumes about the well-developed self-reliant varietal development scenario in the country that caters to all the sugarcane growing zones of the country in terms of improved high yielding, high sugar, stress resistant sugarcane varieties.
The germplasm collection at ICAR-SBI, Coimbatore, has around 4000 accessions. Besides, the National Hybridization Garden (NHG) houses around 500 improved sugarcane clones suited for different parts of India. The Distant Hybridization Facility of ICAR-SBI situated at Agali is also a valuable parental source that contains more than 1200 accessions including Saccharum officinarum clones. These have been well characterized and are being utilized by the sugarcane breeders all over India as parental clones in varietal development. The pre-breeding programmes have contributed improved parental clones to NHG. For example, more than 60 genetic stocks for high sugar and red rot resistance have been contributed by ICAR-IISR, Lucknow, besides the many other improved varieties and superior clones from the other research organizations and SAUs. Many proven crosses involving these improved sugarcane clones/varieties that are developed in India are being carried out by the researchers every year, giving rise to more promising varieties for cultivation in farmers’ field. This is apart from the exotic clones and the World Germplasm Collection housed at the Regional Centre of ICAR-Sugarcane Breeding Institute, Kannur, which has approximately 3300 accessions (ICAR-SBI Annual Report 2020 ). Thus, the country is fully self-sufficient with respect to the improved sugarcane varieties and parental genetic stocks that can be used for genetic base broadening.
The canes bred at SBI, Coimbatore, have spread to several parts of the world. The Coimbatore-developed canes (Co canes) have been reported to be successful in around 28 sugarcane growing countries of the world, either as parental clones for developing location-specific varieties or as commercially cultivated varieties (Amalraj and Balasundaram 2006 ). Thus, the country as a whole has many improved sugarcane varieties with desirable traits, and we are not dependent on other sugarcane growing countries for suitable sugarcane varieties that can satisfy our demand.
Quality Seed Production
Quality seed production is being taken up within the country by the research organizations and also in the farmers’ field. The industry is self-reliant with respect to availability of quality seed of improved varieties, with the participation of the stakeholders. The research institutes under ICAR in collaboration with sugar factories ensure the availability of quality seeds to the growers. Specialised seed nurseries in farmers fields, seed villages for production of quality seeds of improved varieties, entrepreneur development programmes, etc., are some of the strategies that are being taken up to ensure the availability of seeds of improved varieties. ICAR-Sugarcane Breeding Institute, Coimbatore, distributed about 124.5 tons of breeder seed to trained farmers for quality seed production in 2020. Around 1200 tons of quality seed were supplied to the sugar mills of Tamil Nadu. More than 1,62,200 tissue culture raised plantlets were distributed to sugar factories and to the progressive farmers during the year (ICAR-SBI Annual Report 2020 ). ICAR-IISR Lucknow also has a well-established quality seed production programme at Lucknow, and every year approximately 8000 quintals of quality seed of the major sugarcane varieties are being produced and distributed. Besides, entrepreneurship development programmes for quality seed cane production are being conducted regularly wherein the progressive sugarcane farmers are being trained for seed production. Approximately, 20 ha is being brought under this programme annually. With the collaboration of Government of Bihar, a quality seed production programme in sugarcane is being carried out by ICAR-IISR, Lucknow, for making quality seed available to the sugarcane growers of Bihar. An area of 65.0 ha under five sugar mills of Bihar state was earmarked in the first phase, and this initiative has entered its second phase. Around 30,000 quintals of seed of the major sugarcane varieties were produced and distributed to the farmers in Bihar during 2019 (ICAR-IISR Annual Report 2019 ). Such programmes are being carried out by the other R&D organizations also, in areas under their jurisdiction.
Thus, the sugar industry has a well-planned seed production programme within the sugar mill command areas that satisfies the lion-share of seed demand of its stakeholders. Even in the face of unforeseen problems, the sugar mills are in a position to cater to their demand for quality seeds of improved varieties and are not dependent on outside agencies. Simultaneously, the sugarcane growers in these command areas get timely training and scientific inputs from the R&D organizations, and this helps them improve their seed production strategies and methods.
Sugarcane Production Technologies
The sugarcane research institutes have developed many location-specific technologies for improvement in sugarcane and sugar productivity, enhancement in resource use efficiency, irrigation management, profitable intercropping, integrated management of pests and diseases, post-harvest management, etc., which are being used by sugarcane farmers. These are also popularized by Sugarcane Development Departments of states, and various incentives and subsidies are available to farmers. Precision agriculture through site-specific management forms a major component of bio-intensive environment-friendly production package. In the sugarcane production system, it starts right from field preparation and planting, with innovative planting methods and planting materials being utilized.
Modified Planting Material
Single bud planting, primed cane node/bud chip technology and use of other compact forms of planting material in sugarcane, with suitable handling protocols, are cost-effective, with a substantial saving of planting material. Raising settling nursery from any of these starting material and transplanting to the field at the appropriate stage reduces the seed requirement by almost 80%, ensures a good crop stand and thereby good cane and sugar yield. This also reduces the challenges in logistics and transport of the seed material. The technology has been widely adopted, especially in the tropical sugarcane growing zone of the country ( https://www.thehindubusinessline.com/economy/agri-business/bud-chip-technology-catching-on-among-sugarcane-farmers/article30502981.ece ). In the wake of the COVID pandemic, the sugar mills and state cane departments of different states have taken advantage of this technology to a great extent. Women Self Help Groups (SHGs) and other collaborative ventures were formed at the grass root level involving the sugarcane growers in many states like Uttar Pradesh in the sub-tropical India, and the members were imparted training for raising single bud settlings. Around 812 SHGs were constituted across 36 districts in the state of UP and more than 9100 entrepreneurs have been trained. As per official records, using this bud chip technology, till January 2021, 3.51 crore settlings were planted ( https://www.socialnews.xyz/2021/01/09/up-making-women-self-reliant-with-bud-chip-method-of-cane-plantation/ ). Such collective programmes can cater to the demand for planting material in the adjoining sugarcane growing areas, without being dependant on external agencies. This will be cost-effective and can save other resources including labour, besides employment and income generation to these entrepreneurs. Moreover, there will be minimum effect on accessibility to resources even when there are restrictions on mobility and transport and other bottlenecks, as was being witnessed during COVID pandemic. Mechanization for mass production of planting material and use of machinery like settling transplanter for transferring settlings to the field have aided in a better adoption of the technology ( https://sugarcane.icar.gov.in/index.php/en/home/1193-settling-transplanter ; https://sugarcane.icar.gov.in/index.php/en/home/1194-quatro-sugarcane-single-bud-cutter ).
Bio-intensive Nutrient Management
An integrated nutrient management strategy is important in sugarcane, keeping in mind the declining soil health in many of the sugarcane growing areas in India. In many parts of the country, the organic C content in the sugarcane growing soil is low, below 0.4% in some areas. Micronutrient deficiencies have also been reported in these soils. Approximately 9–10 million tons of nutrients (N, P 2 O5, K 2 O) are imported annually to meet the nutrient demand in the agricultural sector as per FAI estimates ( https://www.faidelhi.org/general/Prodn-imp-cons-fert.pdf ). A major share of these chemical nutrients can be substituted with organic sources in an integrated manner. A detailed study conducted in the state of Bihar reported an enhanced B: C ratio of 2.4 with integrated nutrient application over that with the conventional method (1.8) (Thakur et al. 2012 ). Green manuring (including in situ incorporation of green manure crops during land preparation), crop residue incorporation and trash mulching, use of bio-fertilizers and other organics like FYM, sugar industry by-products like bagasse and pressmud are gaining importance in sugarcane too (Shukla et al. 2019 ).
Microorganisms like Gluconacetobacter , Azospirillum , Azotobacter , Pseudomonas , Aspergillus, etc., are some of the microbes that form the components of biofertilizer/microbial consortia. Field trials have indicated that integrating microbial consortium and NPK fertilizer application proved effective in improving soil organic carbon, soil microbial population, microbial biomass carbon, microbial biomass nitrogen, and soil respiration, as well as the cation exchange capacity of the soil (Shukla et al. 2020 ). Several R&D organizations have their own units for large-scale bio-fertilizer production, and these units provide training to the sugarcane growers for mass production of bio-fertilizers. The sugar mills in India also have their own mechanism of bio-nutrient production from industry by-products, and these are supplied to the growers and other stakeholders. There are collaborative linkages among the sugarcane growers, sugar mills and R&D organizations for mass production and distribution of bio-fertilizer and microbial consortia and such linkages help in easy accessibility of the material to the farmers, besides facilitating employment and resource generation. The vast microbial diversity present in the soil and plant parts ensures a steady supply of starting material for large-scale consortia production.
These units established by many sugar mills in their command areas have made the sugarcane growers and other stakeholders self-sufficient in terms of the nutrient requirement, since these organic fertilizers can supplement the chemical fertilizers. Many of the sugar mills and associated research organizations have their own bio-compost units ( http://www.kmsugar.com ; http://www.bannari.com ; https://renukasugars.com ; https://manasindustry.com ; https://www.vsisugar.com ) that make use of sugar factory by-products along with microbial culture to produce their own trademark bio-fertilizers ( Moti Super from KM Sugars, Uttar Pradesh, Bannari Compost from Bannari Amman Sugars, Tamil Nadu, to name a few); thus, the dependency on other sources for these bio-nutrients is done away with. Collective initiatives like out growers’ model of cane cultivation and SHGs can play an active role in such ventures that can overcome many of the challenges in situations where restrictions and regulations are imposed on movement of goods, people and transport. A small portion of the field of selected farmers can be kept apart for bio-composting, and this can serve as a source of organic nutrients to the other sugarcane growers too. Earmarking specific fields in the mill command area itself for growing green manure crops like Sesbania can help in easy availability of seeds. These can be of help in timely green manuring of the fields. Entrepreneurship development for large-scale bio-nutrient production and related management techniques in the field will help in easy accessibility of the technology for the stakeholders and employment generation, along with cost-effectiveness. Even though these initiatives are already being taken up, an intensification of efforts for bio-based nutrient management through establishment of production units and capacity building to facilitate location-specific production at large scale is making the sugar industry self-reliant, even at the grass root level.
Carbon sequestration through trash mulching, rhizosphere engineering and residue incorporation is also an indirect component of nutrient management in this crop which is being practised by sugarcane farmers (Shukla et al. 2017 ). It is estimated that 181 kg C ha −1 yr −1 is sequestered by sugarcane crop (Parr and Sullivan 2005 ). Thus, the practice of growing sugarcane itself leads to maintenance of soil health and enhancement of soil properties, bringing in an element of self-reliance into the whole process of sugarcane cultivation and processing. The regular addition and recycling of plant residues, green manuring and related processes add to this aspect of self-sustenance.
Irrigation Management to Enhance Water Availability
Sugarcane is a crop that requires timely irrigation at the critical stages and a proper management of the available water can help in enhanced water availability. There are several techniques that are being practiced like skip furrow irrigation and micro irrigation systems. It is estimated that out of the total irrigated land in India only less than 20% is under micro-irrigation (Gulati and Mohan 2018 ; https://www.thehindubusinessline.com/data-stories/data-focus/sikkim-andhra-pradesh-karnataka-and-maharashtra-lead-is-usage-of-drip-irrigation/article34075327.ece ). Twenty seven states have only less than 30% of the net cultivated farm lands under micro-irrigation and a few have only 15% of the total cultivated lands under micro-irrigation. Uttar Pradesh the largest sugarcane growing state, has only 1.5% of the land under micro-irrigation. With the present pattern of water consumption, almost half of the demand will remain unmet in 2030. On an average, approximately 32% of water saving can be accomplished with micro-irrigation, with an average income enhancement of 48.5% to the farmer ( https://www.thehindubusinessline.com/data-stories/data-focus/sikkim-andhra-pradesh-karnataka-and-maharashtra-lead-is-usage-of-drip-irrigation/article34075327.ece ). Economic water productivity (EWP) of sugarcane in Bihar and UP is as high as Rs. 36 and Rs. 30 per cubic metre of irrigation water applied, whereas in the tropical states like Maharashtra, Tamil Nadu, Andhra Pradesh and Karnataka, EWP is as low as Rs. 10.7, Rs. 9.3, Rs. 8.3 and Rs. 8.7, respectively, per cubic metre of irrigation water. Similarly, on comparing the economic water productivity across major crops in Maharashtra except sunflower, all other crops produce higher value of output than sugarcane, per unit of irrigation water applied in one hectare (Gulati and Mohan 2018 ). Thus, the use of micro-irrigation systems is necessary in sugarcane especially with the fast depleting ground water table. The situation in states like Maharashtra is an ample proof for this fact. The Government of India implemented Centrally Sponsored Scheme (CSS) on Micro-irrigation in 2006, which was modified on July 1st 2015 to include micro irrigation as a component (per drop more crop) under the PMKSY ( Pradhan Mantra Krishi Sinchayee Yojana ) scheme. At least 10% of the command area created using PMKSY— Har Khet ko pani component—must be covered under micro/ precision irrigation. Other such programmes for water harvesting like Jal Shakti Abhiyaan: Catch the Rain initiative, Atal Bhoojal Yojna are also concerted efforts towards self-sufficiency with respect to water availability.
Apart from the use of micro-irrigation and other agro-techniques, many sugar mills are initiating water harvesting mechanisms in their command/neighbouring areas, that can help in recharging the ground water, at the same time facilitating availability of water for irrigation and other purposes. In a programme on Improving Natural Resources through Integrated Water Resources Management Approach at Sangareddy District, in the Telangana State of South India, Trident sugars, Ltd., has funded a watershed project in which eight water harvesting structures were completed in May 2020, to benefit around 2000 smallholder farmers. The work was supported by ICRISAT, Hyderabad, and also by the International NGO network, Solidaridad. The work continues with more areas being targeted ( https://www.icrisat.org/icrisat-natem-watershed-project-in-south-india-marches-ahead-despite-covid-19/ ). Such initiatives will facilitate availability of water round the year and make the beneficiaries self-reliant with respect to water to a great extent. Another example of such a water harvesting initiative has been reported from Uttar Pradesh, the sugarcane growing belt of sub-tropical India. The distillery division of the Seorahi Chini Mill, Seorahi, Uttar Pradesh, has adopted one pond in a village to initiate rain harvesting ( www.dainikjagran.com ). These are small steps towards making the sugarcane growers and thereby the sugar industry, self-sufficient in water management.
Bio-intensive Crop Protection
The use of inorganic plant protection chemicals has not been very effective in controlling the major diseases and insect pests of sugarcane. Thus, along with the development of resistant/tolerant varieties against the biotic stresses, integrated management including biocontrol of pests and diseases has been an important strategy in this crop. The application of bio-agents for biotic stress control has resulted in a saving of crores of rupees to the government exchequer ( https://ppqs.gov.in ). Bio-agents like Trichogramma, Cotesia and Epiricania are being reared on a large scale and released into the field, and this has been successful against borer complex, Pyrilla , white fly and other pests (Srikanth et al. 2016 ). Trichoderma and other bio-agents have been reported to be effective in managing diseases to some extent, apart from facilitating crop growth (Viswanathan 2018 , 2021 ; Viswanathan and Malathi, 2019 ). Many of the sugar mills and R&D organizations have their own mass rearing facilities for the bio-agents, to rear and release them directly in the fields or to distribute to farmers. Entrepreneurship to facilitate mass-rearing of bio-agents has resulted in training a large number of entrepreneurs in rural areas. These innovative farmers in turn, produce bio-agents on a large scale and distribute it among the growers/stakeholders in a particular area. The rural entrepreneur model of EID Parry is an example (Sankar 2014 ) of how the sugar industry is making use of technology and the available resources to be self-reliant. Apart from the tested bio-agents, the locally available rich biodiversity of Lepidopteron insects/pupae can also be studied for their suitability for use in biological control. This adds another dimension to the concept of a self-sustaining sugar industry.
Mechanization of Sugarcane Agriculture
Perhaps, the most sought after technological advancement that can add to the self-reliance in the sugarcane growing sector, especially in the wake of COVID-19 pandemic-related restrictions, is mechanization, which can supplement the manual interventions in crop production and management. A rough estimate indicates that sugarcane cultivation demands on an average, 375 man hours/ha of the crop (Singh et al. 2020 ). A well-planned and executed mechanization programme in sugarcane cultivation can help in reduction of manual labour with timely and proper field operations, thereby sustaining the crop in the field in adverse situations. This can also minimize human interventions. Even in normal circumstances, mechanization can overcome the labour scarcity and high cost of manual labour, thereby making the cultivation of this labour-intensive crop cost effective. In the wake of second and third waves of the pandemic, at many farms, there would have been the engagement of a new set of labour-force, replacing those who have migrated back to their home-towns and this would also have reduced the efficiency and timeliness of the activities to some extent. Use of machinery has helped to overcome this challenge to a limited level but the extent of mechanization is still not enough for a perfectly smooth and timely conduct of all these operations, in the face of such an unprecedented adversity.
Studies indicate that on an average, the extent of mechanization in sugarcane agriculture is less than 40% in our country, and a lot more needs to be done in this area of adoption of mechanization. But when it comes to the development of machinery, our country has an impressive line-up of R&D organizations ( https://iisr.icar.gov.in ; https://sugarcane.icar.gov.in ) and other engineering groups like M/s Mahindra & Mahindra, Tractors and Farm Equipment Ltd. (TAFE), M/s Deccan Farm Equipments, M/s Eicher Tractors M/s VST Tillers and many others, with a wide range of machinery suited for sugarcane agriculture, ranging from manual and machine operated seed/sett cutters to tractor-operated planters with multiple attachments, RMD, etc., to suite specific needs (Table (Table1). 1 ). The wide range of sugarcane planters and inter-culture equipment from ICAR-IISR, Lucknow, and the sugarcane transplanter, sett treatment device and other equipments from ICAR-SBI, Coimbatore, demand special mention. Manufacturers like M/s Shaktiman have developed sugarcane harvesters for Indian situations, apart from other farm implements ( https://www.shaktimanagro.com/ ). Besides, there are small scale innovators who are engaged in developing equipment like mechanical precision sugarcane sett cutting devices and bud planters ( https://nif.org.in/innovation/sugarcane-bud-planting-machine/796 ). These machines if used extensively in the sugarcane cultivation, have been estimated to result in a saving of more than 60% of manual labour along with a reduction in cost of cultivation to the tune of 50–60%.
Components of self-reliance in Indian sugar industry
The rich resources we have in terms of farm mechanization enable us to be self-reliant in carrying out the various on-farm operations. Indian manufacturers have linkages and collaborations abroad and sugar industries in many countries around the world have taken advantage of the expertise of the manufacturers in sugarcane mechanization. This in turn, has enabled our manufacturers/mechanization experts to sharpen their skills and delve into new avenues, thereby making valuable additions to the Indian mechanization scenario. Thus self-reliance through linkages and collaborations is being realized to a great extent through mechanization, by the Indian sugar industry.
ICT in Sugarcane Agriculture
India is a major sugarcane growing country in the world with different zones having different climatic and other features. Therefore, the requirements for raising a good crop will vary to some extent in these zones. Location-specific packages are needed, and these should be developed in such a way that these are user-friendly. The application of Information & Communication Technology (ICT) has been a significant step towards enhanced productivity and profitability in this crop. This has also facilitated an optimal and timely utilization of resources like human resources, nutrients and water, thereby taking care of the sustainability factor also.
A very significant advancement in the sugar industry has been the GPS-based sugarcane crop area survey initiated by the Indian Sugar Mills Association (ISMA) and other agencies. From 2011 onwards, ISMA has been carrying out sugarcane area survey and estimation using GPS. This helps in timely and more precise estimation and also reduces the requirement of manual intervention. Instead of a minimum of 4–5 personnel needed for sugarcane area survey pre-2011, the GPS-based hand-held machine can work even with a single operator ( http://www.indiansugar.com ).
GPS-based techniques have helped in assessing the location-specific soil nutrient requirements of the sugarcane growers. There are mobile applications developed by different organizations (e.g. Cane Advisor developed by ICAR-SBI, Coimbatore) that are very user-friendly and help in nutrient management. Water management is also one area where the ICT is playing a major role. The mobile-based applications developed help in scheduling irrigation, based on the requirement of the crop. “ Ikshu-Kedar” a mobile-based application for irrigation scheduling, developed by ICAR-IISR, Lucknow is one such intervention ( https://krishi.icar.gov.in ). This, along with proper management practices, helps in timely and need-based irrigation and nutrient management, thereby aiding resource conservation and minimum human interventions. Another such initiative is by the ICAR-Sugarcane Breeding Institute Coimbatore through its Soil Moisture Indicator that has contributed to a highly efficient water utilization by the stakeholders ( https://sugarcane.icar.gov.in/images/sbi/announcements/mobile_app.pdf ). This innovation bagged the National Water Award-2019 for its contribution towards the water conservation.
Several sugar mills have also developed such apps in collaboration with other agencies, e.g. Meetha Sona an interactive App by DSCL Sugars Ltd. ( https://www.amarujala.com/uttar-pradesh/hardoi/meetha-sona-ap-for-sugercane-farmers-hardoi-news-knp5706703180 ). The stakeholders can themselves schedule irrigation in the crop using these applications. Mobile-based diagnostic tools like CaneDES from ICAR-IISR, Lucknow, ‘Plantix’ developed by PEAT, Germany, in collaboration with ICRISAT, India and Acharya NG Ranga Agricultural University, Andhra Pradesh, India, help in disease/disorder diagnosis and management of these stresses ( https://iisr.icar.gov.in/iisr/download/publications/newsletter/NewsLetterJuly2014.pdf ; https://plantix.net ).
Apart from the on-farm ICT solutions, post-harvest activities like marketing have also benefitted immensely from this technology. The Sugarcane Information System (SIS) developed by the Government of Uttar Pradesh is a typical example of how this e-governance platform has made the sale, procurement and marketing of sugarcane easier, along with several other advantages ( https://economictimes.indiatimes.com/news/economy/agriculture/sugarcane-farmers-benefit-through-sis-system-in-uttar-pradesh/articleshow/12019911.cms.from=mdr ; Krishnan 2019 ). The key benefits include transparency in transactions, elimination of middle men, saving of time and resources for frequent travels by the growers, proper weighing of canes and related benefits. Such mobile-based user-friendly applications have been of immense help for sugar industry in challenging times as that was witnessed during the global COVID-19 pandemic. These initiatives are best solutions in overcoming the hurdles of restricted mobility or other such regulations, thereby making the growers also self-reliant.
Process Engineering/Machinery
India has a galaxy of machinery manufacturing and installing companies who have a strong group of extremely capable and experienced technical experts, facilitating an efficient network at the domestic and international level. These manufacturing units are spread all over India and are largely responsible for installation and commissioning of sugar plants, distilleries, co-generation plants and other ancillary facilities for the sugar industry (Table (Table1). 1 ). They constitute some of the world’s leading suppliers of turnkey sugar factories, refineries, distilleries, ethanol plants, power transmission systems, water and waste-water treatment plants, manufacturers of steam turbines, etc. These engineering units have made their mark in installation and commissioning of state-of-the-art sugar industry related facilities both within India and in countries abroad ( https://www.shrijee.com ; https://www.trivenigroup.com ; https://www.isgec.com ; https://thyssenkrup-industries-india.com ; https://www.usipl.com ; https://ssngineers.com and other related groups).With their expertise and vast experience in their field, these companies have a widespread network all over India and also in other countries, where they take up consultancy, installation and commissioning of the latest technological facilities for the sugar industry. To mention a few, the Uttam Sucrotech International Pvt. Ltd., the international arm of Uttam Sugar Group, has projects in Kenya, Indonesia, Sudan, Uganda, Columbia, Ethiopia and many other countries. The Shrijee group has its presence in more than 30 countries across the globe in the form of consultancies, installations and supply of equipment. This is apart from their services that cater to the sugar processing units all over India. The Triveni Engineering Group, ISGEC Heavy Engineering Ltd., SS Engineers, etc., are some of the other sugar machinery and equipment manufacturers who have linkages and collaborations in India and abroad. The list is very lengthy, and the industry has been constantly evolving in this area with newer facilities to meet the ever changing demands of the clientele. The National Sugar Institute, Kanpur, is also having a major role as an advisory and consultancy provider, facilitating linkages among the service providers and stakeholders. The institute provides technical assistance to the sugar mills with an aim to improve their efficiency.
Capacity Building
The entire R&D network of the sugarcane and sugar research organizations are well-equipped with the conventional as well as the modern technologies to provide skill and knowledge enhancement to the students, sugarcane farmers, sugar mill personnel and other stakeholders. Training in sugarcane production and management practices, entrepreneurship development for different technologies like seed production, application of bio-control practices, farm machinery related technologies, skill development in sophisticated techniques including laboratory analyses, molecular biology related techniques, etc., are provided by these organizations as per the demand at regular intervals (Table (Table1). 1 ). During 2019–20220 around 680 farmers, 80 developmental personnel and 570 students were trained at ICAR-IISR, Lucknow (ICAR-IISR Annual Report 2019 ). Such training programmes and interactions are held in other organizations also. Research teams from many sugarcane growing countries like Sri Lanka, Bangladesh, Thailand, Vietnam, Kenya visit these organizations for getting trained in various aspects of sugarcane production and management including mechanization. This is apart from the short visits for specific interactions and deliberations that are regularly organized by these institutions and organization.
Diversification in Sugar Industry: A Step Towards Self-Reliance
Sugarcane and other sugar crops like sugar beet and sweet sorghum are excellent biomass feed stocks for renewable fuel and green energy, apart from several other main and by-products. The major advantage with the sugar crops including sugarcane over the grain crops is the less level of processing required due to the fermentable sugars present in their juice. The fluctuating sugar markets, the demand for alternative bio-based fuels and increased consumer demands for bio-based products are significant factors that drive the global sugar industry towards diversification for sustainability. The continued reliance on fossil fuel energy will deplete the world reserves, and a shift to biomass-based renewable energy will also be environment-friendly, with a reduction in emission of associated greenhouse gases (GHG). With a high biomass and high conversion efficiency, sugarcane has emerged as a very attractive option for diversification to bio-based products. Sugarcane is a major source of bio-energy, and the main energy chains from this crop include bio-ethanol from molasses & juice, cogeneration from bagasse and bio-CNG from press mud and spent wash (Mohan and Kanaujia 2021 ).
Bio-ethanol
In India, there cannot be a more ideal time for the sugar industry to move towards self-reliance, through a sugarcane based bio-economy. A record domestic sugar output in the recent years (32 million metric tons in 2020–21) fluctuating global sugar markets and the demand for green and clean energy, along with the formulation of National Biofuel Policy (NBP) in 2018, has given ample impetus for the sugar industry to divert towards bioethanol. As per the NBP, a blending target of 20% is to be achieved by 2030. The recent reforms in this scenario like E-100 pilot project have led to the government setting new ethanol blending targets ( https://pmindiawebcast.nic.in/2021/5jun21.html ). From the present ethanol blending of approximately 8.5%, a 20% ethanol blending is to be achieved by 2025 and this will drive the diversion of excess sugar/sugarcane juice/molasses towards ethanol production. On June 5, 2021, the Prime Minister of India inaugurated three ethanol dispensing stations at Pune. This E100 pilot project aims to set up a network for ethanol production and distribution across the nation. From around 38 crore litres of ethanol purchased in 2013–14 by the Oil Marketing Companies (OMCs), the amount has increased to 332 crore litres and this approximately eightfold increase in procurement has benefitted the sugarcane farmers to a great extent ( https://pmindiawebcast.nic.in/2021/5jun21.html Sarwal et al. 2021 ). The blending % by PSU OMCs has also increased from 1.53% to 8.5%. With the demand for clean and green energy steadily increasing, the country need to be assured of sufficient supply of bioethanol to meet the future demands in the context of the Ethanol Blending targets. The report of the Expert Committee on Roadmap for Ethanol Blending in India by 2025 , has also highlighted a major role of sugar industry in E 20 blending program ( https://www.niti.gov.in/expert-committee-roadmap-ethanol-blending-india-2025 ). Sugar sector capacity is being augmented to produce 550 crore litres for 20% blending by 2025–26. Molasses-based distilleries can produce 20% additional ethanol if sugar rich feed stocks like B-heavy molasses, syrup and cane juice are used, as the same capacity can cater to the higher demand of ethanol.
Co-generation
Self-reliance in terms of energy demand is vital for the development of any country. India being one among the fastest growing economies of the world, is presently the third largest energy consuming country and is entering a very dynamic period with respect to energy scenario. India has already provided electricity connections to the majority of its citizens, along with the adoption of several energy saving measures. Government has also initiated the massive expansion of renewable energy program of 175 GW by 2022.
Since 2000, India’s per capita energy demand has grown by > 60%. Electricity consumption nearly tripled in the past two decades. The expanding economy and the large scale urbanization and industrialization point towards a huge demand for energy in the times to come. With the co-existence of shortage and abundance of conventional energy sources in the country, there is ample scope of utilization of renewable energy. Co-generation by the sugar mills is an important source that satisfies the energy demand of the country (Mohan and Swain 2018 ; Mohan and Kanaujia 2021 ). Bagasse from sugar processing produces high pressure steam and electricity. The excess power generated after use for running the sugar mill is sent to the national power grid. According to World Alliance for Decentralized Energy, bagasse-based power generation in sugar mills has the potential to meet up to 25% of the present-day power demand in the sugar producing countries of the world.
In India, the total utility electricity sector has about 25% of the total installed capacity being met by newer renewable power plants, which amounts to ~ 94.4 GW (Mohan and Kanaujia 2021 ). 11% of the total installed Renewable Energy Source is contributed by biomass-based co-generation (~ 10 GW). There has been an increase of around 130% in the installed biomass power generation capacity during the last 5 years. This is about 10.15 GW in 2021 ( https://mnre.gov.in ). The sugar industry is an important source of power generation with 275 sugar factories having the facility for co-generation. The current estimate of the exportable energy from sugarcane bagasse from these mills is 3500 MW. This accounts for ~ 1.0% of the total electricity generated in the country. Considering the high-pressure co-generation systems and the latest technologies that are being utilized by the sugar mills, the surplus energy generated can be manifold and this can be exported to the national grid. The present potential of the sugar industry is estimated to be 12.5GW of exportable power generation and this can go up to 14.0 GW with improvements in the infra-structure in the sugar mills (Mohan and Kanaujia 2021 ). The mills will have an improved capacity to produce up to 250 kWh/ton cane against the present generation of around 150 kWh/ton cane. The proper utilization of the sugarcane plant residues in the existing cogeneration units, along with bagasse can help in realizing a higher level of cogeneration in the sugar mills (Mohan and Swain 2018 ). This will help in sustaining the sugar industry and making the industry as well as the country self-reliant in terms of power generation and accessibility to the energy produced.
Bio-gas and Bio-CNG
Compressed bio-gas is another source of bio-energy from sugar industry, which has a significant role in bringing in self-reliance in the energy sector for the country. The anaerobic digestion of filter cake results in the production of bio-gas/compressed bio-gas. Approximately 1 kg of CNG can be produced from 25 to 30 kg of filter cake, which makes the process lucrative. Apart from providing bio-energy this is a value-addition of the industry by-product. Out of the total compressed bio-gas potential of the country (approximately 62.0 million tons), around 3% (around 2.0 million tons) can be produced from sugar industry by-products like spent-wash/filter cake/press mud. Spent wash/vinasse, an effluent from ethanol production process can also be a valuable source of bio-energy. It is estimated that around 500 MW power can be produced from the present available alcohol production (3000 million litres in a year).
Apart from the self-reliance contributed by these value added products, the additional revenue that can be earned by the sugar mills through value addition can contribute to the sustainability of the sugar mills (Table (Table2), 2 ), as estimated in detail (Mohan and Kanaujia 2021 ).
Estimated additional revenue generation through value addition of sugar industry by-products. (Source: Mohan and Kanaujia 2021 )
*Net Revenue considering the additional cost of conversion
Sugarcane can be considered as the best green crude for a bio-based industry, giving rise to an efficient and sustainable bio-based economy. The C 4 route of photosynthesis taking place in the plant, suitability to the tropical environments, ready-to-use source of soluble carbohydrate, the utilization of the whole crop as source of various products, with a very positive energy balance and the lowest carbon foot print, etc., make this crop the best choice for an efficient bio-based economy (Solomon and Swapna 2019 ). Sugarcane provides the raw material for food, fodder, bio-fuel, green energy, bio ethanol, bio-plastics, pulp and paper, particle boards, kitchenware, bio-detergents, bio-molecule coated fabrics, etc. The textiles industry also make use of bagasse based products for manufacturing nature friendly garments). All these contribute towards a self-reliant economy where the dependence on other sources is reduced to a great extent.
Sugarcane-Based Bio-refinery
The biorefinery concept is gaining more importance in India with great investment potential and has been identified as one of the most promising routes for economic sustainability, employment and creation of new industries. The upcoming concept of bio-refinery with sugarcane as the feed stock is an excellent means by which different bio-products can be integrated into the same physical space. The processes for producing sugars, biofuels, co-generation, bio-based products several chemicals (sugar-based or ethanol based) can be undertaken within the same space using different chemistries. The biomass can be converted into high value chemicals that can replace the petroleum-based products. A typical example of a sugarcane based refinery is presented here (Fig. 1 ), and many sugar mills are producing these value added products.

Bio-refinery from sugarcane—options for Indian sugar industry
An excellent example of product diversification into bio-based chemicals while obtaining social, environmental, and financial sustainability gains, is Godavari Biorefineries Ltd., in India ( https://godavaribiorefineries.com/ ). Starting with sugarcane, Godavari manufactures refined sugar, ethanol, and chemicals including ethyl acetate, crotonaldehyde, 1,3-butanediol, and even flavour and fragrance ingredients. From bagasse, electricity is cogenerated and used to power the sugar and chemicals factories and plants. The company’s bio-based chemicals are produced so efficiently that they are able to compete pricewise against the same chemical produced from fossil sources ( https://godavaribiorefineries.com/ ). Many sugar companies such as The Simbhaoli Sugar Mills, Mawana Sugar works, Harinagar Sugar Mills, Balrampur Chini Mills and Bajaj Hindustan, Ltd., are using sugarcane and its co-products and employing cleaner chemical processes to make environmentally preferred products to satisfy consumers who are becoming increasingly aware of sustainability issues.
The Indian sugar industry like other progressive sugar producing countries is increasingly implementing sustainable ways to produce and supply natural sweeteners (Eggleston and Lima 2015 ) such as cane sugar, jaggery, unrefined raw/brown sugar, syrups following a circular approach which spans the entire product life cycle.
Sugarcane Industry and Carbon Footprints
Several studies have been carried out all over the world., esp., in the sugarcane growing countries like Brazil about the sustainable green technologies in terms of CO 2 emissions, carbon and water footprints, methane emissions, etc., that can be adopted in sugarcane agriculture. Sugarcane cultivation and processing practices as a way towards reduction of carbon foot prints has assumed great significance in the present scenario of environmental friendly sustainable options. Land management, nutrient management, plant residue incorporation and green harvest are some of the practices which have grabbed the attention of researchers in this regard (Solomon and Swapna 2019 ). Estimation of C footprint in the sugar production chain has been reported under different situations in major sugar producing countries/regions like Brazil, Australia and Philippines (Rein 2010 ; Renouf et. al. 2010 ; de Oliveira Bordonal 2018 ; Mendoza 2014 , 2017 ). In India, sugarcane-based cropping system (Sugarcane-Ratoon-Wheat) was observed to sequester 1.42 mg/ha/yr carbon and with the residue incorporation along with application of Trichoderma , a sequestration up to 2.45 mg/ha/yr of carbon could be achieved (Shukla et al. 2017 ). A longer sustainability of soil C level, crop productivity and production efficiency was documented by the group under these conditions. BONSUCRO through its Better Sugarcane Initiative Standards have also estimated net carbon footprint for sugar production. As a base value, within specific conditions and with the assumption of 10 MW power exported from co-generation, an estimated value of 307 kg CO 2 equivalent/ton of sugar (0.31 g CO 2 eq/g of sugar) produced has been reported by this group. Hiloidhari et al. ( 2021 ) identified some of the conditions that can lead to better environmental friendly production practices in Maharashtra in India. The carbon emission/carbon footprint values are found to depend on the country/ region of study and the variables used in the estimations. Increasing the efficiency of agricultural operations in the field and processing in the factories using bio-intensive practices will invariably lead to reduced carbon footprint.
Transition towards a “Green Smart Sugar-Agro Complex” with an emphasis to produce green energy and other bio-based products, apart from sugar is the hallmark of the present-day sugar factories. Improved technologies like GREEN technology (Greatly Reduced Energy and Equipment Needs) for sugar refining (Tongaat Hulett Refinery, South Africa) are being practiced by the sugar mills. The potential of value addition through a better utilization of by-products is also being taken up by the sugar mills, for example, bagasse for co-generation, molasses as a source of ethanol, utilization of vinasse/spent wash for bio-electricity and also for soil health management, to name a few. A bio-refinery with the mandate of “Wealth from waste” encourages the sugar mills to utilize “anything and everything from the sugarcane plant” to be processed for an enhanced profitability and sustainability of the industry (Fig. 1 ).
There have been investigations related to carbon footprint of bioethanol and bioelectricity production from sugarcane. A comparison of carbon footprints from US sugarcane bioethanol and Brazil sugarcane bioethanol by Mekonnen et al. ( 2018 ) reported that the Brazil bioethanol from sugarcane has a smaller carbon foot print (38.5 kg CO 2 eq/MJ) than that of US corn bioethanol (44.9 kg CO 2 eq/MJ).
The carbon foot print of bioelectricity production from sugarcane bagasse was estimated to be 0.227 kg CO 2 eq./KWh compared to that of 1.660 kg CO 2 eq/KWh electricity from diesel (Carvalho et al. 2019 ). Here also, the main contributors of CO 2 in the sugarcane production process were concluded to be fertilizer applied and irrigation.
The Indian sugar industry has been making use of these strategies both in the field as well as the factory in an attempt to lower the carbon foot prints. Several incentives and directives are in place to facilitate such sustainable activities aimed at reducing the carbon-footprint from the sugar industry. Of late, in the state of Uttar Pradesh, the government has mandated a 33% of afforestation through adopting Japanese Miyawaki technique, which is expected to bring about a major curb in the carbon emissions ( https://timesofindia.indiatimes.com/city/lucknow/uttar-pradesh-asks-industries-to-adopt-miyawaki-method-to-reduce-carbon-footprint/articleshow/87027089.cms ). Such bio-intensive production and management techniques, carbon sequestration, micro-irrigation practices, integrated nutrient and pest/disease management, green harvesting, product diversification and other energy efficient processing practices are all a part of our efforts towards a reduction in carbon foot-prints.
Indian Sugar Industry and the 2030 Agenda for Sustainable Development
The 2030 Agenda for Sustainable Development Goals and its 17 Sustainable Development Goals (SDGs), provide a visionary road map for all countries and stakeholders to strive for a world of sustainable prosperity, social inclusion and equality, while at the same time preserving the planet and leaving no one behind. It embraces three dimensions of sustainability—economic, social and environmental. In order to carry forward the common vision of a sustainable, peaceful and prosperous future, sugar industry is gradually transforming toward Self-reliance to Sustainability so it can leverage new opportunities created by global shifts in sugar production and trade due to Covid-19 pandemic, as well as new options of renewable energy (ethanol, electricity, CNG), and bio-based products (bio-plastics, bio-chemicals, wellness products) through bio-refinery concept (Table (Table3). 3 ). The industry has taken necessary sustainability initiatives for all its key stakeholders, resources, environment and product development through various Government departments, agencies, NGOs, CSR, etc. Many companies are implementing ISO certification/BSI/Bonsucro Production Standard—a global, metric-based standard for improving the social, environmental and economic sustainability of sugarcane farming and sugarcane derived products. Such standards are useful in strengthening the sugar mill efforts to get its sugar cane suppliers to adopt sustainable farming practices while helping it to improve workplace safety and monitoring. This has greatly helped in empowering small holders to get better cane yields through bio-intensive sugarcane production technologies, better and efficient use of resources, recycling and value addition of products, and production & economic sustainability.
Agenda 2030 for Sustainable Development—Contribution by the Indian sugar industry
Farmer Sustainability
The Indian sugar industry has a vision to create sustainable value for the farmers by improving resource efficiency, revitalizing ecosystems and uplifting rural communities. In this regard, smallholder farmers face the challenges of low returns and therefore industry is committed to teach them techniques about new & alternative ways of income generation through enhancing cane productivity, entrepreneurship and intercropping. Experts from research institutes, KVKs and NGOs share knowledge and expertise in sustainable agriculture practices in sugarcane cultivation, improved varieties, water management, biological control methods, bio-fertilizers, mechanization, marketing and pre- and post-harvest management of produce which limit any negative impact on the environment. Many progressive units also provide tools and training that make their farms more productive, efficient and profitable while preserving the long-term potential of their land for a sustainable livelihood.
Resource Sustainability
The i ndustry is committed and equipped to manufacturing products using bio renewable raw materials and then ensuring that the full value of raw materials is maximized. This starts with the planning of efficient manufacturing processes that enable economic sustainability for the sugar mills through higher product yields and environmental sustainability using fewer resources and low emissions and waste. All residues and co-products (bagasse, molasses, PMC and cellulosic residues) are used as feedstock for another value added product throughout the value chain (bio-fuels/bio-electricity/biochemicals/bio-plastic/pharmaceuticals/feed/fodder/paper/boards/moulded products).The spent wash generated in distilleries burnt in an incinerator boiler helps in meeting the steam and power requirements of the distillery. Additionally, the ash from the boiler is used to make bricks, further benefiting to reduce the environmental pollution and disposal problem & more importantly reduces the cost of construction. The rest of the spent wash is used in the manufacturing of bio compost by utilizing press-mud received from sugar mill (using aero-tillers), thereby leading to zero liquid discharge.
Environmental Sustainability
Indian sugar industry have overcome various challenges to become second largest producer of sweeteners in the world, however, in process of doing so, significant negative impacts were inflicted onto the environment, esp . on to the water resources. To overcome these impacts and to promote sustainable practices, Central Pollution Control Board (CPCB) of India introduced a charter and environmental compensation plan for the sugar industries. The CPCB guidelines is being followed in all the sugar & distillery units which has resulted in significant reduction in freshwater consumption, effluent generation, and biochemical oxygen demand load. Besides, adoption of rainwater harvesting practices, recycling of PMC through bio-composting and CNG production, use of sludge for the purpose of filling low lying areas, and consumption of used oil with bagasse in boilers are other significant moves in the line of environmental sustainability and economical operation of sugar industries after the implementation of charter. The outcome of these initiatives has been very effective in minimizing the pollution load from sugar industries and in conserving the groundwater resources and promoting environmental sustainability. Our sugar mills and distilleries have adopted Zero Liquid Discharge (ZLD) system, an advanced wastewater treatment technology to recycle, recover and re-use the treated wastewater and effluent, ensuring no discharge of wastewater to the environment. Green energy sources like bio-ethanol, bio-electricity and bio-CNG are being promoted by Government of India through use of molasses, cane juice, bagasse, biomass and PMC. There are as many as 275 bagasse based power co-generation plants which are designed to meet their own power requirements as well as export the surplus power to grid, providing much needed power to the community and villages nearby. This process actually mitigates carbon emissions, and the industry earns Carbon Credits under the United Nations Framework Convention on Climate Change (UNFCCC).
Sustainable Valorisation
The sustainability strategy is an inherent part of sugar industry business plan and consistent with its commitment to contribute to the society. The sugar industry is an enabler of improved sustainability across value chains and manufactures many bio-based products through value addition. The industry is producing a range of renewable products that not only have lower environmental impact but also perform equivalent or superior to existing materials. The use of resources to make green power (bio-ethanol and bio-electricity), that also mitigate greenhouse gases, and the manufacture of biofuels and chemicals from ethanol also presents a model as to how a crop such as sugarcane can be used to make a host of valuable products, used in a variety of applications. Recent EBP of Government of India, where sugar industry is supplying a major chunk of ethanol shows that fossil fuels have an alternative and the green fuel produced from sugarcane is an examples of this model. The industry R & D is engaged in developing innovative ways for further valorizing residual biomass by cascading which includes the recovery of silicon from bagasse ashes, production of bio-plastic and bio-fertilizer, and valorization of biogenic CO 2 sources.
Social Sustainability
All the key stakeholders directly involved in sugar value chains viz., farmers, farm workers, sugar mills, cane societies, Cane Development and Sugar Industry Departments, companies buying sugar, civil societies and trade unions are directly or indirectly associated with the sugar units of their area. Sugarcane production in the command area is the direct responsibility of cane development department wherein a large number of farmers including women farmers are associated. Sugar mills operate many technology-enabled cane collection centres across the villages and provide convenient transportation and logistics to the farms of the partner farmers, so that the agricultural produce can be transported with ease and no added costs are incurred by the farmers. This service enables farmers to concentrate on their production activities while the industry take care of the aggregation of their agricultural produce. The harvested sugarcane is subsequently transferred to the manufacturing and processing units. The sugar mills are responsible for taking care of the farming community as per the Companies Act, 2013 by implementing CSR policy. The CSR activities of sugar mills are focused on different sectors with main emphasis on promotion of education, skill development, health care and preventive healthcare, gender equity and empowering women (Solomon et al. 2019 ). During Covid-19 pandemic, the industry has worked closely with government hospitals, schools, police stations and other establishments to assist in the fight against the pandemic. Many mills had arranged sanitizers, facilitated company ambulance for shifting affected patients, arranged for RT-PCR/random tests and provided other required services.
The global markets are in a transition phase with a relentless drive to improve the efficiency along with sustainable development. Rapid growth and growing prosperity has created an increase in demand for all the resources, including food, feed and energy. A lion-share of this demand is invariably coming from the Asian countries, with the fast growing economies like India poised to take a quantum leap with respect to economic growth. Thus, the Asian countries and the South East Asian region as a whole, are the leading players in driving the global economic growth. With a fast developing economy, the demand for food, fuel, feed and other products and resources will be more, which necessitates an increase in availability of these resources. This calls for a judicious utilization of resources along with the need for self-reliance. The unforeseen challenges like the ongoing pandemic also offer ample scope to restructure the industry with a minimum level of dependence on other sources with collaborations and linkages to strengthen the self-sufficiency. The sugar industry is a perfect example of an emerging sustainable system with ample avenues to become self-reliant. The innovative technologies in the sugarcane and sugar production system along with the scope for diversification has made the global as well as the local sugar industries, the flag bearers of the concept of sustainability and profitability with self-reliance. This is evident from the remarkable growth of the Indian sugar industry from the early 30 s till date and its transformation into a major sugar producing nation with a strong global presence. The Indian sugar industry in particular, is far ahead in this journey and has the potential to lend a helping hand to the sugar producing countries of the region so that the sugar industry in the Asia and South East Asian Region can be trailblazers in their march towards a self-reliant, sustainable, bio-based economy.
For the Indian sugar industry, this journey towards self-reliance and sustainability is a continuous one, taking along with it the various stakeholders, investors, employees and other clientele, for the welfare and economic prosperity of the overall community.
Declarations
Authors declare that they have no conflict of interest.
Publisher's Note
Springer Nature remains neutral with regard to jurisdictional claims in published maps and institutional affiliations.
Contributor Information
S. Solomon, Email: moc.liamg@2591lihsusnomolosrd .
M. Swapna, Email: moc.liamg@anpawsragus .
- Amalraj VA, Balasundaram N. Status of sugarcane genetic resources in India. PGR Newsletter FAO-Bioversity. 2006; 148 :26–31. [ Google Scholar ]
- Anonymous. 2021. Indian Sugar . LX XII (3): 39–45.
- Carvalho M, Da Silva Segundo VB, De Medeiros MG, Dos Santos NA, Coelho ML., Jr Carbon footprint of the generation of bioelectricity from sugarcane bagasse in a sugar and ethanol industry. International Journal of Global Warming. 2019; 17 (3):235–251. doi: 10.1504/IJGW.2019.098495. [ CrossRef ] [ Google Scholar ]
- de Oliveira Bordonal R, Carvalho JLN, Lal R, de Figueiredo EB, de Oliveira BG, La Scala N., Jr Sustainability of sugarcane production in Brazil. A Review. Agronomy for Sustainable Development. 2018; 38 :13. doi: 10.1007/s13593-018-0490-x. [ CrossRef ] [ Google Scholar ]
- Eggleston G, Lima I. Sustainability issues and opportunities in the sugar and sugar-bio-product industries. Sustainability. 2015; 7 :12209–12235. doi: 10.3390/su70912209. [ CrossRef ] [ Google Scholar ]
- Gulati, A., and G. Mohan. 2018. Towards sustainable, productive and profitable agriculture: Case of Rice and Sugarcane. Working Paper No. 358. Indian Council for Research on International Economic Relations (ICRIER), New Delhi.
- Hiloidhari M, Banerjee R, Rao AB. Life cycle assessment of sugar and electricity production under different sugarcane cultivation and cogeneration scenarios in India. Journal of Cleaner Production. 2021; 290 :125170. doi: 10.1016/j.jclepro.2020.125170. [ CrossRef ] [ Google Scholar ]
- http://www.bannari.com
- http://www.indiansugar.com
- http://www.kmsugar.com
- https://economictimes.indiatimes.com/news/economy/agriculture/sugarcane-farmers-benefit-through-sis-system-in-uttar-pradesh/articleshow/12019911.cms?from=mdr Accessed from 10 Nov 2021.
- https://godavaribiorefineries.com/
- https://iisr.icar.gov.in/iisr/download/publications/newsletter/NewsLetterJuly2014.pdf
- https://krishi.icar.gov.in
- https://manasindustry.com
- https://nif.org.in/innovation/sugarcane-bud-planting-machine/796 Accessed from 16 Nov 2021.
- https://plantix.net
- https://pmindiawebcast.nic.in/2021/5jun21.html Accessed from 5 Jun 2021.
- https://renukasugars.com
- https://sugarcane.icar.gov.in/index.php/en/home/1193-settling-transplanter Accessed from 20 Nov 2021.
- https://sugarcane.icar.gov.in/images/sbi/announcements/mobile_app.pdf
- https://sugarcane.icar.gov.in/index.php/en/home/1194-quatro-sugarcane-single-bud-cutter Accessed from 20 Nov 2021.
- https://www.thehindubusinessline.com/economy/agri-business/bud-chip-technology-catching-on-among-sugarcane-farmers/article30502981.ece Accessed from 25 Oct 2021.
- https://www.thehindubusinessline.com/data-stories/data-focus/sikkim-andhra-pradesh-karnataka-and-maharashtra-lead-is-usage-of-drip-irrigation/article34075327.ece Accessed from 29 Oct 2021.
- https://www.amarujala.com/uttar-pradesh/hardoi/meetha-sona-ap-for-sugercane-farmers-hardoi-news-knp5706703180 Accessed from 10 Nov 2021.
- https://www.faidelhi.org/general/Prodn-imp-cons-fert.pdf Accessed from 2 Nov 2021.
- https://www.icrisat.org/icrisat-natem-watershed-project-in-south-india-marches-ahead-despite-covid-19/ Accessed from 29 Oct 2021.
- https://www.jagran.com Accessed from 5 Nov 2021.
- https://www.socialnews.xyz/2021/01/09/up-making-women-self-reliant-with-bud-chip-method-of-cane-plantation/
- https://www.vsisugar.com
- ICAR-IISR Annual Report. 2019. ICAR-Indian Institute of Sugarcane Research, Lucknow-226 002. Uttar Pradesh.
- ICAR-SBI Annual Report. 2020. ICAR-Sugarcane Breeding Institute, Coimbatore-641 007. Tamil Nadu.
- Krishnan BS, Gupta P, Yadav R, Ramawat N. Sugar Information System: A pro-farmer initiative in Uttar Pradesh. Plant Archives. 2019; 19 (1):1285–1291. [ Google Scholar ]
- Mekonnen MM, Romanelli TL, Ray C, Hoekstra AY, Liska AJ, Neale CMU. Water, energy, and carbon footprints of bioethanol from the U.S. and Brazil. Environmental Science & Technology. 2018; 52 :14508–14518. doi: 10.1021/acs.est.8b03359. [ PubMed ] [ CrossRef ] [ Google Scholar ]
- Mendoza TC. Reducing the carbon footprint of sugar production in the Philippines. International Journal of Agricultural Technology. 2014; 10 (1):289–308. [ Google Scholar ]
- Mendoza TC. No burning sugarcane trashes makes sugarcane production - net carbon sequestering. International Journal of Agricultural Technology. 2017; 13 (2):247–267. [ Google Scholar ]
- Mishra VK. Performance of sugar industry in India during pre and post-independence period. International Journal of Creative Research Thoughts. 2018; 6 (1):450–460. [ Google Scholar ]
- Mohan, N., and D. Swain. 2018. Efficient use of sugarcane bioenergy for sustainability of Indian sugar industry. Journal of Bioremediation Biodegradation 9: 74 10.4172/2155-6199-C1-014
- Mohan N, Kanaujia AK. Bio-energy from Indian sugar industry: A sustainable renewable energy future. International Journal of Engineering Research & Technology. 2021; 10 (5):990–996. [ Google Scholar ]
- Parr JF, Sullivan L. Soil carbon sequestration in phytoliths. Soil Biology and Biochemistry. 2005; 37 (1):117–124. doi: 10.1016/j.soilbio.2004.06.013. [ CrossRef ] [ Google Scholar ]
- Ram B, Hemaprabha G. The sugarcane Co 0238: A reward to farmers and an elixir to sugar industry. Current Science. 2020; 18 (11):1643–1646. [ Google Scholar ]
- Rein PW. The carbon foot-print of sugar. Sugar Industry/Zuckerindustrie. 2010; 135 (7):427–434. doi: 10.36961/si10006. [ CrossRef ] [ Google Scholar ]
- Renouf M, Wegener MK, Pagan RJ. Life cycle assessment of Australian sugarcane products with a focus on sugarcane growing. The International Journal of Life Cycle Assessment. 2010; 15 (9):927–937. doi: 10.1007/s11367-010-0226-x. [ CrossRef ] [ Google Scholar ]
- Sankar M. Exploitation of Biocontrol Agents, Trichogramma chilonis and Tetrastichus howardi on Yield Improvement in Sugarcane at EID Parry (India) Ltd., Sugar Mill Command Areas. International Journal of Innovative Research and Development. 2014; 3 (8):314–318. [ Google Scholar ]
- Sarwal, R., S. Kumar, A. Mehta, A. Vardan, S.K. Singh, S.S.V. Ramakumar, and R. Mathai. 2021. Roadmap for ethanol blending in India 2020–2025 . NITI Aayog India Ministry of Petroleum and Natural Gas, Government of India.
- Shukla SK, See S, Maity SK, Solomon S, Awasthi SK, Gaur A, Pathak AD, Jaiswal VP. Soil carbon sequestration and crop yields in rice-wheat and sugarcane–ratoon–wheat cropping systems through crop residue management and inoculation of Trichoderma viride in Subtropical India. Sugar Tech. 2017; 19 :347–358. doi: 10.1007/s12355-016-0470-x. [ CrossRef ] [ Google Scholar ]
- Shukla SK, Zubair A, Awasthi SK, Pathak AD. AICRP technical publication-II. Lucknow: ICAR-All India Co-ordinated Research Project on Sugarcane, ICAR-IISR; 2018. Sugarcane varieties identified by AICRP (S) in India. [ Google Scholar ]
- Shukla SK, Solomon S, Sharma L, Jaiswal VP, Pathak AD, Singh P. Green technologies for improving cane sugar productivity and sustaining soil fertility in sugarcane-based cropping system. Sugar Tech. 2019 doi: 10.1007/s12355-019-00706-z. [ CrossRef ] [ Google Scholar ]
- Shukla SK, Sharma L, Jaiswal VP, Pathak AD, Tiwari R, Awasthi SK, Gaur A. Soil quality parameters vis-a-vis growth and yield attributes of sugarcane as influenced by integration of microbial consortium with NPK fertilizers. Scientific Reports. 2020; 10 :19180. doi: 10.1038/s41598-020-75829-5. [ PMC free article ] [ PubMed ] [ CrossRef ] [ Google Scholar ]
- Singh S, Singh PR, Singh AK. Status of sugarcane mechanization in India. In: Singh S, Singh AK, Pathak AD, editors. Improved sugarcane mechanization technologies. India: ICAR-Indian Institute of Sugarcane Research, Lucknow; 2020. p. 177. [ Google Scholar ]
- Sinha OK. Fortyfive f AICRP on sugarcane. Lucknow: All India Co-ordinated Research Project on Sugarcane, ICAR-Indian Institute of Sugarcane Research ; 2016. [ Google Scholar ]
- Solomon S. Sugarcane agriculture and sugar industry in India: At a glance. Sugar Tech. 2014; 16 :113–124. doi: 10.1007/s12355-014-0303-8. [ CrossRef ] [ Google Scholar ]
- Solomon S, Rao GP, Swapna M, Kumar A, Singhal RC. Corporate social responsibility initiatives and their impact on sugar-mill performance: A case study of the Seksaria Biswan Sugar Factory, India. Proceedings of the International Society of Sugar Cane Technologists. 2019; 30 :377–383. [ Google Scholar ]
- Solomon S, Rao GP, Swapna M. Impact of COVID-19 on Indian Sugar Industry. Sugar Tech. 2020; 22 :547–551. doi: 10.1007/s12355-020-00846-7. [ PMC free article ] [ PubMed ] [ CrossRef ] [ Google Scholar ]
- Solomon, S., and M. Swapna. 2019. Green technologies in sugarcane agriculture: Mitigating climate change. IAPSIT Newsletter 1 & 2: 2–3.
- Srikanth, J., S. Easwaramoorthy and S.K. Jalali. 2016. A 100 years of biological control of sugarcane pests in India: Review and perspective. CAB Reviews 11 (013): 1–32.
- Thakur SK, Jha CK, Alam MM, Singh VP. Productivity, quality and soil fertility of sugarcane ( Saccharum spp complex hybrid) plant and ratoon grown under organic and conventional farming system. Indian Journal of Agricultural Sciences. 2012; 82 (10):896–899. [ Google Scholar ]
- Viswanathan R. Changing scenario of sugarcane diseases in India since introduction of hybrid cane varieties: Path travelled for a century. Journal of Sugarcane Research. 2018; 8 (1):1–35. [ Google Scholar ]
- Viswanathan R, Malathi P. Biocontrol strategies to manage fungal diseases in sugarcane. Sugar Tech. 2019; 21 :202–212. doi: 10.1007/s12355-018-0690-3. [ CrossRef ] [ Google Scholar ]
- Viswanathan, R. 2021. Red rot of sugarcane ( Colletotrichum falcatum . Went). CAB Reviews 16 (023): 1–57.
Accessibility Links
- Skip to content
- Skip to search IOPscience
- Skip to Journals list
- Accessibility help
- Accessibility Help
Click here to close this panel.

Purpose-led Publishing is a coalition of three not-for-profit publishers in the field of physical sciences: AIP Publishing, the American Physical Society and IOP Publishing.
Together, as publishers that will always put purpose above profit, we have defined a set of industry standards that underpin high-quality, ethical scholarly communications.
We are proudly declaring that science is our only shareholder.
Water-food-energy challenges in India: political economy of the sugar industry
Ju Young Lee 1 , Rosamond L Naylor 1,2 , Anjuli Jain Figueroa 1 and Steven M Gorelick 1,3
Published 24 July 2020 • © 2020 The Author(s). Published by IOP Publishing Ltd Environmental Research Letters , Volume 15 , Number 8 Citation Ju Young Lee et al 2020 Environ. Res. Lett. 15 084020 DOI 10.1088/1748-9326/ab9925
You need an eReader or compatible software to experience the benefits of the ePub3 file format .
Article metrics
7450 Total downloads
Share this article
Author e-mails.
Author affiliations
1 Department of Earth System Science, Stanford University, Stanford, CA 94305, United States of America
2 Center on Food Security and the Environment, Stanford University, Stanford, CA 94305, United States of America
3 Woods Institute for the Environment, Stanford University, CA 94305, United States of America
- Received 24 March 2020
- Accepted 3 June 2020
- Published 24 July 2020
Peer review information
Method : Single-anonymous Revisions: 1 Screened for originality? Yes
Buy this article in print
Sugar is the second largest agro-based industry in India and has a major influence on the country's water, food, and energy security. In this paper, we use a nexus approach to assess India's interconnected water-food-energy challenges, with a specific focus on the political economy of the sugar industry in Maharashtra, one of the country's largest sugar producing states. Our work underscores three points. First, the governmental support of the sugar industry is likely to persist because policymakers are intricately tied to that industry. Entrenched political interests have continued policies that incentivize sugar production. As surplus sugar has been produced, the government introduced additional policies to reduce this excess and thereby protect the sugar industry. Second, although the sugar economy is important to India, sugar policies have had detrimental effects on both water and nutrition. Long-standing government support for sugarcane pricing and sales has expanded water-intensive sugarcane irrigation in low-rainfall areas in Maharashtra, which has reduced the state's freshwater resources and restricted irrigation of more nutritious crops. Despite its poor nutritional value, empty-calorie sugar has been subsidized through the public distribution system. Third, the Indian government is now promoting sugarcane-based ethanol production. This policy has the benefit of providing greater energy security and creating a new demand for surplus sugar in the Indian market. Our analysis shows that a national biofuel policy promoting the production of ethanol from sugarcane juice versus directly from molasses may help reduce subsidized sugar for human consumption without necessarily expanding water and land use for additional production of sugarcane.
Export citation and abstract BibTeX RIS
1. Introduction and approach
India is the second largest producer and largest consumer of sugar in the world [ 1 ]. With decades of various government supports, the sugar industry is highly regulated and the second largest agro-based industry (after the textile industry) in India [ 2 ]. The industry plays an important role in the Indian economy, accounting for 1% of the national GDP and supporting over 6 million farmers plus numerous workers in harvest, transport, distribution, and wholesale [ 3 ]. Furthermore, India has over 550 sugar mills and closely related secondary industries [ 3 ].
India now faces a sugar paradox: on one hand, the government supports the production of both sugarcane and sugar with financial incentives and price supports; on the other hand, the government spends billions of dollars to remove surplus sugar. These conflicting actions, resulting from the political economy of the Indian sugar industry, have led to interconnected water-food-energy challenges experienced nationally and locally in major sugar producing Indian states. Local impacts are exemplified in Maharashtra, one of the leading sugar-producing states, which produces about one-third of India's sugar and 5% of the world's sugar (2010–2019 average) [ 1 , 4 ]. In Maharashtra, expansion of water-intensive sugarcane depletes the state's water resources and reduces irrigation and production of other nutritious crops. Meanwhile, the government subsidizes empty-calorie sugar against the country's nutritional goals. The government also promotes sugarcane-based bioethanol, which can enhance energy independence but also further strain water and land resources by expanding production of sugarcane feedstock.
Over the past decade, research on the water-food-energy nexus has been conducted in many areas of the world and is now widely accepted as a field of study to build new understanding of the interconnectivity among these three resources [ 5 – 8 ]. This field of research provides critical knowledge for coordinated management to improve the security and sustainability of water-food-energy systems [ 5 – 7 ]. Although water-food-energy nexus studies often assess the role of government policies on resource use, few focus on the influence of the political economy—that is, how policymakers have entrenched economic interests in the industry they govern [ 9 ]. Existing studies provide partial accounts of the water-food-energy nexus in India based on the role of minimum support prices for wheat and rice and flat-tariffs for agricultural electricity; together, the studies demonstrate how those policies lead to unsustainable use of groundwater, over-production of those crops at the expense of high-value and more nutritious crops, and acute financial instability of electric utilities [ 10 – 13 ].
There has been no study that comprehensively assesses the water-food-energy challenges specific to India's sugar industry in the context of its political economy. Academic literature has addressed the political economy of the sugar industry in Maharashtra and in India [ 14 – 18 ] but not its relationship with water-food-energy security holistically. The media and NGOs often attribute water security problems in Maharashtra to the control of water by politicians who own sugar mills [ 19 – 21 ]; however, their analysis is incomplete because it does not address the connections with food and energy security or the more complex ways that the government has supported the industry.
Our approach to studying water-food-energy challenges in Maharashtra consists of two methods. First, we use the nexus approach that examines the latest available data and literature to arrive at new insights into the links, feedbacks, and tradeoffs within the water, food, and energy system. Second, we analyze the water-food-energy dynamics through a political economy lens, which incorporates a careful review of the history of political and economic incentives surrounding the sugar industry, as well as an assessment of contemporary policies [ 22 – 24 ]. Combining these approaches permits us to develop a narrative around the water-food-energy nexus; although lacking causal attribution, this approach provides an economic and behavioral context for understanding the persistent challenges across India's resource sectors.
As shown in figure 1 , this paper discusses the following major themes: what interconnected water, food, and energy challenges arise from the sugar industry and how the political economy drives the challenges. Table 1 summarizes the spatial scales used and our rationale for their selection. We use specific spatial scales that are suitable for analysis of the political economy and of each challenge. Although using a single spatial scale would simplify the exposition, it would fail to capture some linkages that exist at different spatial scales. Section 2 assesses the nexus challenges and discusses the role of the political economy (sub-section details in figure 1 ), and section 3 concludes with main take-aways from this paper and future directions for this line of research.
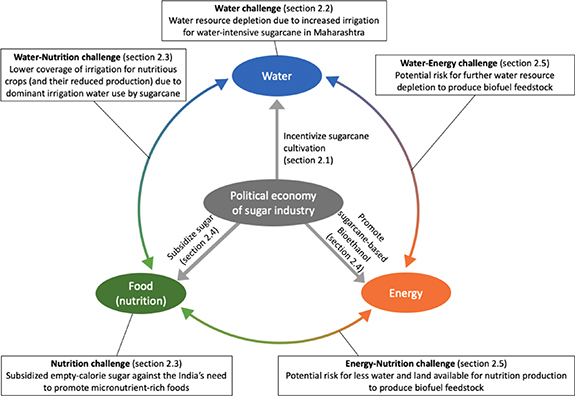
Figure 1. Water-food-energy nexus driven by the political economy of the sugar industry in India. Government policies supporting the sugar industry contribute to regional water depletion, poor nutrition, and biofuel expansion that relies on scarce land and water resources.
Download figure:
Table 1. Spatial scales for the nexus challenges and political economy analyzed in this paper. Water-food-energy challenges are experienced at different spatial scales, and the government policies driving the challenges are at national and state levels. This paper discusses each challenge and the political economy at the suitable spatial scale.

2. Water-food-energy challenges and political economy
2.1. government supports for the sugar industry driving water and nutrition challenges.
India's sugar industry, which took off under government protection in 1932, has rapidly grown under the long-standing government supports from the 1950s through the present. The government has used various interventions to support the entire value chain of the sugar industry: guaranteed sale 1 and minimum price for sugarcane, financial assistance to sugar mills (especially cooperatives), monthly releases of sugar in free markets to regulate sugar price (abolished in 2012), and subsidized sugar provided to consumers [ 14 ]. These government supports for the sugar industry, which were initiated to enhance rural development after Indian Independence was achieved in 1947, have persisted due to consolidation of political power during the sugar industry's growth.
Maharashtra presents an excellent example of how the sugar industry gained political power. Since the 1950s, the state's policies favored the promotion of sugar cooperatives [ 15 ]. These cooperatives enabled sugarcane farmers to become shareholders in their regional sugar mill. The cooperatives played a critical role in rural development by creating employment at sugar mills and subsidiary industries as well as building schools, colleges, and hospitals [ 16 ]. As sugar cooperatives became important to the rural economy, their board chairmen and directors, who were elected by cooperative member farmers, gained considerable prestige and patronage [ 17 ]. With the status and power of sugar cooperative board members, major political parties began recruiting them into local and state politics [ 17 , 18 ]. In turn, sugar cooperatives made contributions to political election campaigns of their board members, and those elected became 'sugar baron' politicians, who continued government support for the sugar industry [ 18 ].
Overall, sugar mills and politicians created a symbiotic relationship that had a reinforcing cycle [ 15 ]. This relationship, especially between sugar cooperatives and the Congress Party is evident: 74% of the chairmen of sugar cooperatives were elected as members of the Legislative Assembly and Parliament during the 1952–1972 period [ 17 ]. This strong rural support from the sugar cooperatives has enabled the Congress Party to dominate state politics in Maharashtra [ 17 ]. Since the late 1990s, some financially unhealthy cooperative mills were liquidated and converted to private mills, and new private mills were additionally established [ 18 , 25 , 26 ]. However, these private sugar mills are also in the hands of the 'sugar baron' politicians who either own or indirectly control them [ 18 ].
Figure 2 shows that the number of sugar mills and sugar production in Maharashtra have rapidly increased since the 1950s. 2 Over this period, the Congress Party was in power except for short interruptions and introduced various supports for the sugar industry. Figure 3 shows that the districts with a larger sugar industry tend to show stronger support for the Congress Party.
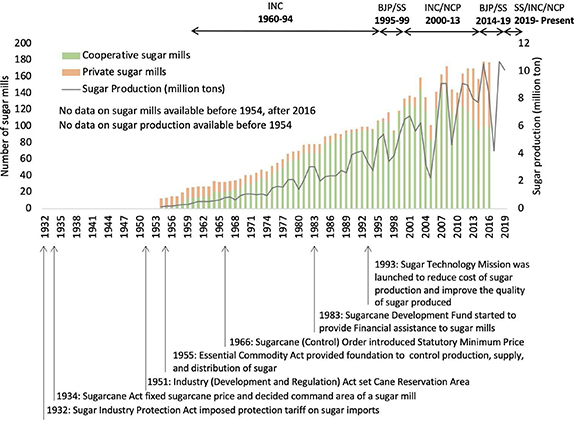
Figure 2. Interconnectedness between major ruling party in Maharashtra state government, the number of cooperative and private sugar mills (under operation) and sugar production in Maharashtra, and key supports/legislation to support the sugar industry. Since the Maharashtra government was established in 1960, the Congress Party (INC) alone (1960s–1990s) or with its splinter NCP (2000s–2010s) has mostly dominated the state government and continued the legacy of government support for the sugar industry that started in 1932. Under continued government support, sugar production and the number of sugar mills in Maharashtra have increased on trend since the 1950s. Political party acronyms: INC: Indian National Congress (the Congress party); NCP: Nationalist Congress Party; BJP: Bharatiya Janata Party; SS: Shiv Sena. Note on political parties: Having split from the INC in 1999, the NCP was also led by strong sugar interests and often formed an alliance with the INC. In contrast to INC and NCP, which have developed a symbiotic relationship with sugar mills and strong vote base in rural areas, BJP and SS have a traditional vote base in urban areas. In recent years, BJP and SS are also making inroads to rural areas developing connections with the sugar sector. Source: Sugarcane production from [ 4 , 25 , 26 ]; Number of sugar mills from [ 25 , 26 ]; Key supports/legislation from [ 27 ]; Major ruling party from [ 28 ].
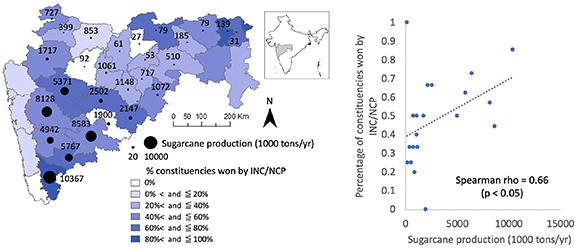
Figure 3. District-level relationship between the level of sugarcane production (average of 1998–2018) and the support for the INC. (Indian National Congress; the Congress Party) or its splinter NCP (Nationalist Congress Party) in the 2019 Legislative Assembly election in Maharashtra: (a) on the Maharashtra district map and (b) in a scatter plot. Districts with major sugarcane production (>130 000 tons per year, which is the lowest quartile) tend to support the INC. or NCP. Both parties are led by strong sugar interests and often form a political alliance. Source: Sugarcane production from [ 29 ]; Author's aggregation of the 2019 election results at constituency level from [ 28 ] to district level (supplemental material 1 (available online at stacks.iop.org/ERL/15/084020/mmedia ) for constituency level data).
To date, the strong sugar lobby from the coalition of sugarcane farmers, sugar mills and 'sugar-baron' politicians ensures continued support for the sugar industry, including a minimum price and guaranteed sales of sugarcane to sugar mills. As a result, sugarcane has become the most profitable crop in Maharashtra [ 30 ] (supplemental material 2). Based on our informal interviews with farmers and local agricultural experts, it appears that there are several reasons in addition to high returns why farmers prefer sugarcane: sugarcane requires less labor, can be grown in soil with some degree of toxicity (e.g. heavy metals), and can withstand longer watering intervals. 3 However, sugarcane's stable high profitability, particularly relative to alternative crops, remains an important incentive for its cultivation.
2.2. Water resource depletion
Sugarcane is a water-intensive perennial crop, and the varieties grown in Maharashtra require 1700–2400 mm irrigation per growth cycle of 11–17 months [ 31 ]. Sugarcane has expanded particularly in dry Western Maharashtra, taking advantage of irrigation infrastructure and electricity subsidies for pumping groundwater. Because the basaltic (hard-rock) aquifer in Maharashtra has limited groundwater storage [ 32 ], groundwater resources alone are often insufficient to irrigate sugarcane. Therefore, sugarcane is grown in or near cultivable command areas (CCAs), 4 tapping primarily surface water from canals, rivers, and reservoirs, and secondarily groundwater (figure 4 ). Decades of rapid growth of sugarcane cultivation and irrigation have increased pressure on the state's water resources.
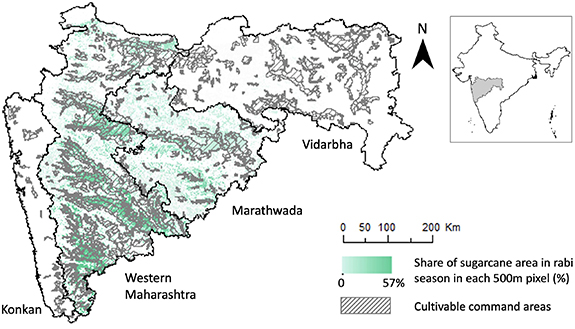
Figure 4. Sugarcane cultivated area in the rabi (winter) season in Maharashtra in 2005. Sugarcane is mainly cultivated in areas with canal water access in Western Maharashtra. Source: Sugarcane cultivated area in the rabi (winter) season in Maharashtra in 2005 (the latest year with available data at pixel level) from [ 33 ]; Cultivable command areas in Maharashtra from National Hydrology Program in India.
In 2010–11, sugarcane, which occupied only 4% of total cropped areas, consumed 61% of irrigation water use. 5 Indeed, during the past half century, sugarcane production in Maharashtra has increased seven-fold from an average of 11 million tons in the 1960s [ 25 ] to 75 million tons in most of the 2010s (2010–2018) [ 29 ], mainly through expanding areas of sugarcane cultivation (supplemental material 3). Along with area expansion, sugarcane has continued to rely on flood irrigation [ 34 ], thereby leading to a rapid increase in irrigation water use. From 1970–71 to 2010–11 irrigation water use by sugarcane has increased faster than any other crop and dominated irrigation water use in Maharashtra (figure 5 ).
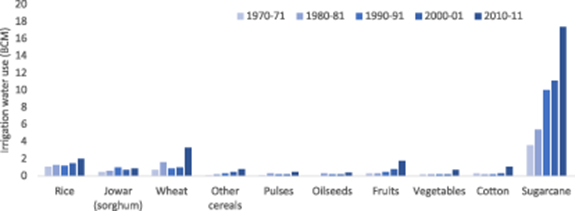
Figure 5. Irrigation water use by major crops or crop groups in Maharashtra from 1970–71 to 2010–11. In Maharashtra, irrigation water use by sugarcane has increased more rapidly than any other crop over time, and sugarcane has used the highest share of total irrigation water in all time periods. Source: Authors' calculations (supplemental material 4 for data, assumptions, and calculations).
Geographically, farmers cultivate sugarcane mainly in low-rainfall areas of Western Maharashtra, taking advantage of surface water storage and canal irrigation infrastructure. Such infrastructure, which was initially established in Western Maharashtra to fight droughts and famines in the early 20th Century [ 35 ], opened opportunities for irrigated agriculture and allowed expansion of sugarcane there [ 15 , 35 ]. Due to the perishable nature of sugarcane, sugar mills were developed there as well. As the sugar mills gained political connections, Western Maharashtra benefited from a higher rate of irrigation development, which further enabled sugarcane cultivation [ 36 ].
In addition, sugarcane farmers also disproportionately benefit from electricity subsidy for groundwater pumping. This agricultural subsidy was implemented in Maharashtra in 1977 as a flat tariff mainly to improve billing efficiency [ 37 ]. At present, unmetered agricultural pumps get charged a flat rate tariff based on the pump capacity, and metered agricultural pumps are charged a subsidized volumetric rate tariff lower than the production cost of electricity. 6 While the subsidy encouraged use of electricity for both surface water and groundwater pumping, it particularly encouraged groundwater use, giving farmers more access to and control of water. The subsidy substantially reduced the irrigation costs, which was particularly beneficial for water-intensive sugarcane. Also, the comparatively well-off sugarcane farmers were financially better able to drill wells and invest in irrigation infrastructure (i.e. pumps) and take advantage of subsidized electricity [ 37 ].
Rapid expansion of sugarcane and dominant water use to irrigate sugarcane in the low-rainfall areas has raised concerns regarding water scarcity and allocation in Maharashtra. In 1999, the Maharashtra Water and Irrigation Commission recommended that sugarcane should be banned in areas that received less than 1000 mm rainfall per year [ 38 ], and many water experts have repeated similar recommendations [ 3 ]. Nevertheless, 82% of sugarcane cultivation falls in regions with low-rainfall (<1000 mm/year) where farmers have access to surface water irrigation (figure 6 ).
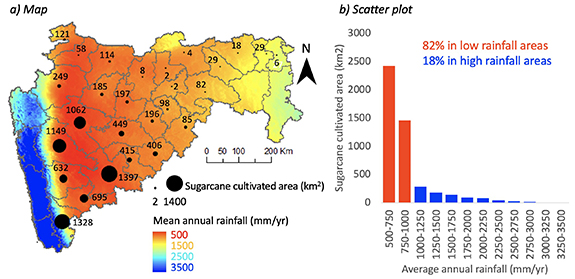
Figure 6. Comparison of mean annual rainfall to sugarcane cultivated area: (a) district-level sugarcane cultivated area (average of 2007–2018) on the Maharashtra rainfall map (average of 2007–2018) and (b) sum of pixel-level sugarcane cultivated area (2005; 500 m resolution) by rainfall levels in a bar plot. Sugarcane is mostly grown in low-rainfall districts in Maharashtra that have less than 1000 mm rainfall per year, which is the minimum annual rainfall for sugarcane cultivation recommended by the government. Note that the Western Ghats, a mountainous range, creates the large disparity in rainfall distribution between the westernmost districts and the rest of the state. More details about our analysis for this figure and additional analysis of sugarcane production versus rainfall can be found in supplemental material 5. Source: District-level sugarcane cultivated in 2007–2018 from [ 27 ]; Sugarcane cultivated area in the rabi (winter) season in Maharashtra in 2005 from [ 33 ]; Map of mean annual rainfall in 2007–2018 from [ 39 ].
To analyze the hydrologic impact of sugarcane, we examine the case of the Upper Bhima Basin. The region is a watershed of great interest because it has significant area planted with sugarcane, and data from the Basin show hydrologic impacts of increasing sugarcane irrigation. From 2000 to 2010, the Upper Bhima Basin saw a substantial increase in irrigation water use for sugarcane and a marginal increase for other crops (figure 7 (a)). Accordingly, the Basin's outflow was reduced from 1996 to 2012 (figure 7 (b)). Our analysis of groundwater heads during 1996–2016 shows no statistically significant long-term decline in groundwater levels in the Basin (supplemental material 6). However, over the long term, groundwater levels are likely to decline once a new hydrologic equilibrium is reached, which can take decades. Currently, the impact of groundwater depletion appears as a reduction in baseflow of rivers and short-term drying of wells during droughts.
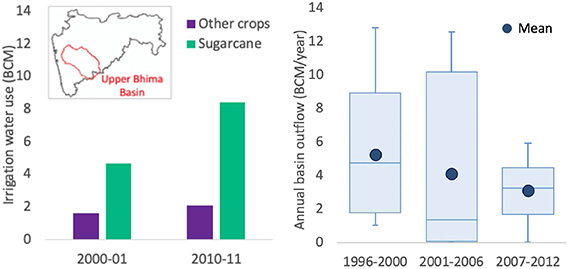
Figure 7. Analysis of the Upper Bhima Basin: (a) irrigation water use by sugarcane and other crops in 2000–01 and 2010–11 and (b) annual river outflow averaged over three time periods (1996–2000, 2001–2006, and 2007–2012) showing a similar precipitation level (36, 35, and 37 BCM, respectively). In the Upper Bhima Basin, sugarcane irrigation has substantially increased from 2000–01 to 2010–11, while irrigation has marginally increased for other crops. As sugarcane irrigation has increased, the average river basin outflow has decreased over the similar period. Source: (a) Irrigation water use: author's calculations (supplemental material 4 for data, assumptions, and calculations); (b) river outflow: data from the basin simulation office located in Pune, Maharashtra.
2.3. Reduced production and consumption of nutritious crops
India's leading nutritional challenges today are protein and micronutrient deficiencies [ 40 , 41 ]. Micronutrients are required in small amounts but essential to human health; their deficiency can lead to illness, disabilities, and even death [ 42 ]. India faces micronutrient deficiency in iron, vitamin A, vitamin B12, vitamin C, folate, iodine, zinc, and copper [ 41 , 43 ]. For example, the most common form of micronutrient deficiency in India, anemia caused by iron deficiency, afflicts roughly 50% of preschool children and contributes to 70% of maternal deaths [ 41 , 44 , 45 ].
The sugar industry worsens nutrition insecurity in two ways. First, our work in Maharashtra shows that the dominant water use to cultivate sugarcane prevents irrigation and production of other crops that contain protein and micronutrients. Although Maharashtra has the largest number of dams in India [ 46 ], much of the developed irrigation potential is being diverted to cultivate sugarcane. This diversion, in turn, has forced less water-consumptive, nutritious food crops largely into rainfed areas, showing much lower irrigation coverage than the national average. Cereals and pulses grown in Maharashtra generally require 300–800 mm of water [ 47 ], which is still less than the water requirement for sugarcane when adjusted for their shorter growth periods. Nonetheless, as of 2013–14, irrigation coverage of cereals and pulses was only 22% and 12% in Maharashtra, compared to 60% and 20%, respectively, in India overall (figure 8 ). Only 19.6% of Maharashtra's cropped area is irrigated, less than half of the national average of 47.7% [ 48 ]. Consequently, nutritious food crops, left to be grown under rainfed conditions, not only suffer from low yields but also remain vulnerable to variability in the timing and intensity of monsoons [ 48 , 49 ]. Although households can purchase protein and micronutrient-rich food produced in other states, local production is important for household nutritional outcomes, especially in remote rural areas in India where transportation infrastructure and supply chains are poorly developed [ 50 , 51 ].
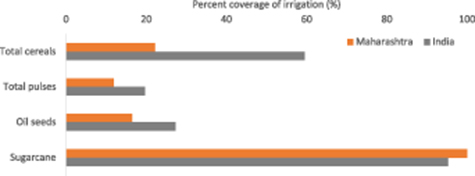
Figure 8. Percentage coverage of irrigated area under principal crops, 2013–14. In Maharashtra, cereals, pulses, and oilseeds are significantly less irrigated than the national average in order to irrigate water-intensive sugarcane. Source: [ 48 ].
The second way that the sugar industry affects nutrition is that the Indian government encourages sugar consumption by providing it at a subsidized price through the public distribution system (PDS). 7 Sugar provides high but 'empty' calories with no nutritional value (figure 9 ). Since the 1950s, sugar has been subsidized through the PDS in India [ 52 ]. At that time, India had experienced frequent famines, and the most pressing food security challenge was to meet basic calorie requirements [ 53 ]. In that context, PDS sugar may have been beneficial in providing cheap calories. Since then, India has greatly improved its provision of calories through growth in wheat and rice production associated with the Green Revolution in the 1960s and 70s and with subsequent agricultural policies [ 53 ]. In today's India, calorie intake is broadly sufficient across income groups, but micronutrient deficiency remains a widespread problem [ 53 ]. Nonetheless, PDS continues to distribute subsidized sugar but does not include other crops high in micronutrients and protein such as pulses and legumes. Given that the PDS provides an incentive to purchase relatively low-cost sugar over more expensive, nutritious crops outside of the system, the program does not solve India's current nutrition challenge. Instead, the PDS helps to support sugar demand, indicative of the sugar industry's strong political influence.
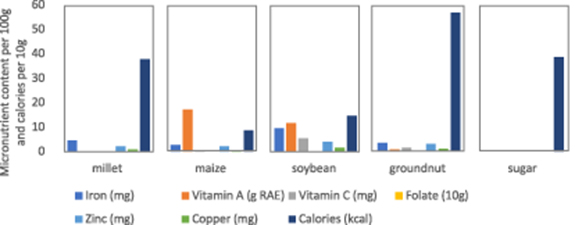
Figure 9. Micronutrient content and calories of sugar and selected crops. Sugar provides empty calories with no nutritional value. Source: Micronutrient content from [ 54 ] and calories from [ 55 ].
The PDS shifted from a universal system to one that targets only the poor in 1997 [ 52 ] but still distributes a large amount of sugar to a number of beneficiaries at a large social cost. In 2016–17, the PDS provided about 2.7 million tons of sugar to 400 million beneficiaries throughout India or approximately 30% of the country's total population [ 56 ]. Over the same period, the Indian government spent around 45 billion rupees (640 million USD 8 ) on sugar subsidies for the PDS, accounting for 4.3% of total food subsidies [ 57 ]. Between 2004–05 and 2011–12, sugar consumption rose for lower income groups, mostly from the increase in their PDS sugar consumption [ 58 , 59 ]. Although other factors also contribute to India's overall increasing sugar consumption (e.g. rising GDP per capita and greater consumption of sugary drinks), the PDS sugar program continues to play an important role at the expense of public financial resources and the nutrition of lower income groups.
2.4. Sugarcane-based ethanol production
In addition to electricity subsidy for groundwater pumping, another link of energy to the water-nutrition challenge is sugarcane-based ethanol production. India's long-term dependency on foreign oil continues to rise with escalating fuel demands as the economy develops [ 60 ]. By 2030, India's population will reach 1.5 billion, and the country is expected to have 430 million vehicles on the road [ 61 ]. To increase its energy self-reliance, the Indian government launched the Ethanol Blending Petrol (EBP) Program in 2003 [ 62 ]. Not coincidentally, this policy was introduced at the time when the sugar industry began to face new financial challenges.
After decades of growth, India's sugar industry faced substantial surplus sugar production and increasing sugar stocks by the 1990s (figure 10 ). Because the Indian sugar industry is highly subsidized, the domestic sugar price in India has consistently been higher than the world sugar price, making India's surplus sugar uncompetitive in the international market (supplemental material 7) [ 3 ]. This surplus sugar has led to the decline in the real sugar price in India (supplemental material 7). Meanwhile, the agricultural sector's share of income and labor in India has declined due to more balanced growth in non-agricultural sectors resulting from urbanization and industrialization. At this stage, the Indian government had two options on the sugar industry's future: either 'reduce' or 'protect' it. Due to vested political and economic interests in the sugar industry, the government has chosen the latter approach, consistent with the process of structural transformation experienced in virtually all large agricultural producing countries [ 63 ]. While continuing to support over-production of sugarcane and sugar, the government has adopted several measures to remove the surplus, such as subsidizing sugar exports and promoting PDS sugar. Introducing sugarcane-based ethanol through the EBP served the dual purpose of enhancing energy security and propping up the sugar industry by providing a diversified source of income to sugar mills and removing surplus sugar.
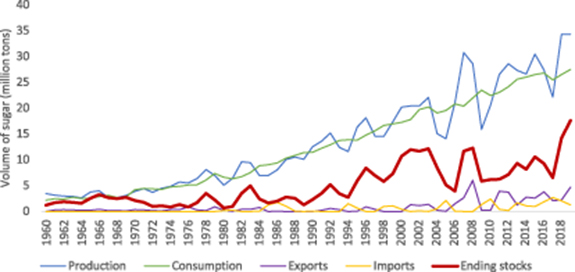
Figure 10. Sugar stock growth and production, consumption, and trade trends in India (1960–2018). Sugar stock in India has substantially increased from the 1990s (see footnote 2 for the cyclical fluctuations of sugar production). Source: [ 1 ].
2.5. Risks and opportunities of sugarcane-based ethanol in India
There are many examples in India and around the world of energy policies negatively affecting water and food resources. The electricity subsidy for agriculture in northern India has depleted groundwater, threatening food production [ 13 ]. Corn-based biofuel in the United States has inflated the price of corn for food and fodder [ 64 ], and ethanol policies in Brazil have incentivized the expansion of sugarcane cultivation at the expense of some staple food crops [ 65 ]. In India, sugarcane-based ethanol may also worsen the water and nutrition challenges highlighted above by requiring more water and land resources to cultivate additional sugarcane. There may be an opportunity, however, to limit the water-food downsides of bioethanol in India. If implemented properly, diverting sugar to ethanol production could improve energy self-reliance and reduce subsidized sugar consumption without having to increase sugarcane cultivation.
Under the EBP, the mandatory blending rate of ethanol with gasoline for public Oil Marketing Companies (OMCs) increased from E5 (5% ethanol) in 2008 to E20 (20% ethanol) in 2017 [ 66 ]. The highest blending rate achieved by 2017, however, was 3.3% (E3) due to insufficient ethanol production capacity, competing demands for potable and industrial ethanol, and noncompetitive pricing for fuel ethanol [ 67 ]. In 2018, the government extended the E20 target year to 2030 [ 68 ]. By 2030, India's gasoline demand is projected to increase to 94 billion liters of pure gasoline (figure 11 ). Given that ethanol provides 40% less energy content (density) than gasoline [ 69 ], when the two are blended more of this blended fuel is required than pure gasoline to provide the same total energy. Meeting India's gasoline demand in 2030 will require 101 billion liters of E20 blended gasoline, which will require 20 billion liters of ethanol, an increase of over eight times its current production. To reach this target, the government has introduced several new measures, such as providing financial assistance to sugar mills to enhance ethanol production capacity and raising fuel ethanol prices for the OMCs [ 69 ].
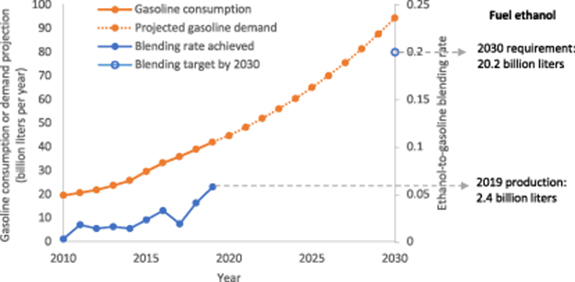
Figure 11. Gasoline consumption (2010–2019) and demand projection (2020–2030) with ethanol-to-gasoline blending rate achieved (2010–2019) and targeted by 2030. The government is aiming to increase the ethanol-to-gasoline blending rate from the current 5.8% to 20% by 2030. Since gasoline demand is projected to grow fast by 2030, achieving the target blending rate of 20% will require a substantial increase in ethanol production. Source: Gasoline consumption and blending rate in 2010–2019 from [ 70 ]; Gasoline demand projection for 2020–2026 from [ 71 ]; Gasoline demand projection for 2027–2030 extrapolated using the same percentage increase from the 2020–2026 data.
The most important measure introduced recently allows the use of sugarcane juice in ethanol production, in addition to molasses (a by-product from sugar processing with less extractable sugar remaining 9 ), which was permitted previously [ 68 ]. This measure has enabled the sugar industry to divert some sugarcane juice from sugar production to ethanol production. To incentivize such diversion, the government has also set differential pricing for ethanol produced from molasses and sugarcane juice, where the price of ethanol from sugarcane juice is set higher [ 69 ]. As a result of these measures, the blending rate of ethanol grew to 5.8% in 2019, but ethanol was still largely produced from molasses [ 70 ]. 10 It requires investments and time, however, for distilleries to modify ethanol production to use sugarcane juice as a feedstock. Also, the availability of sugarcane juice for ethanol production is affected by sugar demand in any given year. Though the share of raw materials in ethanol production in future years is somewhat unpredictable, ethanol production is likely to grow under the government measures aimed at achieving E20 by 2030.
Although E20 is a desirable goal for improved energy security, India's biofuel policy should also be assessed for its impacts on water-food challenges. In particular, it is important to ask: How much sugarcane is required to meet India's biofuel E20 mandate by 2030, and what will be the consequences for water resources and nutrition? To assess the potential range of such impacts, we consider two future scenarios of ethanol production: continuing the current practice of heavily relying on molasses or entirely switching to sugarcane juice.
The first scenario of continuing to rely on molasses presents significant risks to India's water and nutrition challenges through additional demand placed on sugarcane. Meeting E20 with ethanol produced from molasses by 2030 would require an additional 1320 million tons of sugarcane, 348 billion m 3 of water, and 19 million hectares of land in India (figure 12 ); or approximately 385% increases over their respective current level (2010–2018 average) assuming current average yields and water use rates for sugarcane in India (see supplemental material 8). These increases in water and land resources for sugarcane could intensify competition for these resources for nutritious crops and would further deplete water resources. Although it is possible to grow the additional sugarcane in new areas where water resources are not constrained, the political interests already established in Maharashtra and other major sugarcane producing states would likely keep sugar production mainly in those regions. At the same time, the increased sugarcane supply would lead to production of an additional 161 million tons of sugar, which is only slightly less than the world's current sugar output. Since adding this much sugar to the international market would cause the sugar price to plummet, the Indian government would need to further subsidize Indian sugar to be exported or consumed via the PDS. If put into the PDS, the extra sugar produced could encourage more consumption of empty-calorie sugar, which counters India's nutritional challenge of micronutrient deficiency.
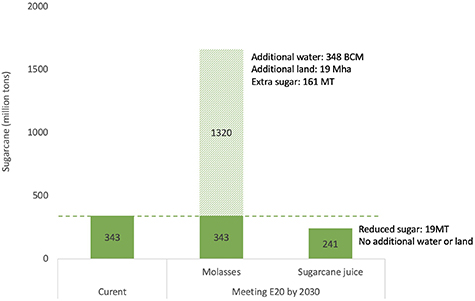
Figure 12. Meeting E20 by 2030: additional sugarcane, water and land resources needed, and extra sugar produced. Meeting the 20% ethanol-to-gasoline blending rate by 2030 with ethanol produced from molasses would require additional water and land resources and produce extra sugar. In contrast, ethanol produced from sugarcane juice could meet the blending target without risking water and land resources and would reduce extra sugar. Source: Authors' calculations (supplemental material 8 for data, assumptions, and calculations).
The other scenario of ethanol production from sugarcane juice presents an interesting possibility of meeting E20 without having to increase sugarcane supply and with the nutritional benefits of reducing PDS sugar. The current level of sugarcane production is sufficient to supply the amount of ethanol needed to meet E20 by 2030 if ethanol comes entirely from sugarcane juice (figure 12 ). Therefore, water resource depletion and competition for water and land resources for nutritious crops would at least not worsen further. In addition, using sugarcane juice for bioethanol could reduce sugar production by 19 million tons per year, which is more than the current stock of surplus sugar in India. By removing some of this surplus sugar, the government would be able to reduce spending on its export subsidy and PDS sugar. Nevertheless, a reduction in government spending would likely be minimal, since subsidies for ethanol development would be required. However, removing surplus sugar could help reduce the amount of subsidized PDS sugar and promote consumption of more nutritious crops that are needed to attain nutrition security in India. Such nutritional benefits would not occur if ethanol were made predominantly from molasses.
3. Discussion and conclusion
To summarize, we have shown how the sugar industry in India presents interconnected water-food-energy challenges that are locally experienced in Maharashtra, a major sugar-producing state, and more broadly in India. Our analysis adds to the existing literature on India's water-food-energy nexus, which focuses primarily on wheat and rice, and demonstrates how the political economy of the sugar industry perpetuates distortions in all three resource sectors. Focusing on the political economy dimensions of the water-food-energy nexus helps to clarify the root causes and future trajectory of India's resource challenges.
Our paper highlights three main conclusions. First, entrenched political interests have long supported the sugar industry in Maharashtra through various policies, including guaranteed prices and sale for sugarcane and public distribution of sugar. Policies supporting the sugar industry are unlikely to disappear in Maharashtra, since the policymakers and the 'sugar barons' are often one and the same. Second, although the sugar industry has played an important role in the Indian economy, government sugar supports have had widespread repercussions in the water and food sectors. Price supports and guaranteed sales to sugar mills have incentivized farmers to expand cultivation of water-intensive sugarcane in low-rainfall areas in Maharashtra, depleting the state's freshwater resources and restricting irrigation of more nutritious crops. By maintaining its subsidized sugar through the public distribution system, the government has also promoted the consumption of empty-calorie sugar. And third, a new policy for sugarcane-based bioethanol, if implemented wisely, could enhance energy self-reliance and reduce subsidized sugar for human consumption. Specifically, using sugarcane juice as opposed to molasses as a feedstock for ethanol could help meet energy targets without requiring additional water and land resources for sugarcane production. This approach would likely reduce surplus sugar and eliminate the need for public distribution of sugar.
Future research is needed to develop a systems model to quantify and simulate water-food-energy interactions of the sugar industry in India. Such a model can be used to evaluate future policy options that point the way for India to achieve water-food-energy sustainability. Our study provides a useful foundation for development of such a policy evaluation model by providing insights regarding which policy options might be feasible given the entrenched political and economic interests in the industry. Key to model utility is a deep understanding of the political economy that enables a more realistic analysis of future trajectories of interconnected water-food-energy systems.
Acknowledgments
This work was supported by US National Science Foundation (NSF) grant ICER/EAR1829999 to Stanford University under the Belmont Forum Sustainable Urbanisation Global Initiative (SUGI)/Food-Water-Energy Nexus theme. Any opinions, findings, and conclusions or recommendations expressed in this material do not necessarily reflect the views of the NSF. We also thank Yoshihide Wada, Sudatta Ray, Philip Womble, Abigail Nora Birnbaum and Steven Seungkyun Ko for providing valuable comments.
Data availability statement
The data that support the findings of this study are available from the corresponding author upon reasonable request. Summary streamflow data is presented in the paper, and raw streamflow data can be obtained upon request from the Basin Simulation Office located in Pune, Maharashtra in India.
Through Cane Area Reservation that puts sugar mills and sugarcane farmers in a contractual relationship to ensure adequate supply of sugarcane to sugar mills.
The cyclical swings in sugar production are caused by natural factors such as droughts and pest problems and also induced by government regulations. Government regulations supporting sugarcane production result in a glut of sugarcane and sugar production, thereby depressing the sugar price. This lower sugar price leads to reduced profitability for sugar mills and delayed payments to sugarcane farmers. As the sugarcane arrears increase, farmers switch to other crops. Then, sugarcane and sugar production decreases, followed by higher sugar prices, higher profitability and lower arrears, and the cycle repeats [ 3 ].
Sugarcane's tolerance to water stress is important given the high variability in seasonal rainfall and canal water operations.
Cultivable command area is defined as the area which can be irrigated from canals and is fit for cultivation.
The Report on Sugarcane Price Policy in 2014–15 states that sugarcane consumes 71.5% of total irrigation water in Maharashtra [ 32 ], but their calculation does not include fruits and vegetables. See supplemental material 4 for more details for our calculations.
The Maharashtra State Electricity Distribution Co. Ltd. serves 3.7 million Agriculture consumers, out of whom approximately 2.2 million are unmetered [ 37 ].
The PDS distribute food and non-food commodities, such as wheat, rice, sugar, and kerosene, to India's poor at subsidized rates [ 52 ].
For this calculation, the average exchange rate in 2019, 71 Indian rupees/USD, is used.
Two types of molasses are used for ethanol production: molasses-C and molasses-B. Molasses-C is a final by-product from sugar processing with no economically extractable sugar remaining, and molasses-B is an intermediate by-product with some extractable sugar remaining.
Out of 2400 million liters (ML) of bioethanol produced in 2018–2019, 1800 ml (75%) was produced from molasses-C, 420 ml from molasses-B (18%), 20 ml (1%) from sugarcane juice, and 160 ml (7%) from damaged food grains [ 66 ].
Supplementary data (1.33 MB, PDF)
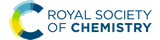
Sugarcane bagasse based biorefineries in India: potential and challenges

First published on 10th November 2020
Sugarcane bagasse (SCB) is one of the world's most abundant agricultural residues and in an Indian context, ∼100 million tonnes per annum is produced. The current use of SCB is restricted to the cogeneration of steam and power; however considering its potential, cogeneration is not the best valorisation route. Furthermore, with falling electricity prices and reducing global sugar prices due to excess sugar stock, it is inevitable that the waste generated (SCB) by sugar mills are utilised for generating revenue sustainably. With this background, this review aims to put forth a biorefinery perspective based on SCB feedstock. Biogas and bioethanol are the Government of India's current focus with policies and subsidies clearly pointing towards a sizeable future market. Therefore, alongside these biofuels, high-value chemicals such as xylitol, succinic acid and lactic acid were identified as other desired products for biorefineries. This review firstly discusses SCB pre-treatment options based on end applications (saccharification or anaerobic digestion, AD). Next, state-of-the-art for each of these aspects was reviewed and our perspective on a profitable biorefinery is presented. We propose an AD based biorefinery where vortex-based hydrodynamic cavitation was found to be the best choice for pre-treatment. AD is considered not only a bioprocess for energy production here but also a ‘pre-treatment’, where partial conversion of holocellulose leads to a digestate rich in a loosened fibre matrix. This digestate rich in cellulose can be enzymatically hydrolysed and further valorised biochemically. This approach would be cost effective and provide a sustainable waste management route for sugar mills.
Introduction
While this increased the overall wellbeing of sugarcane farmers, the growth of the sugarcane industry also led to associated waste related issues. The major solid waste streams generated in the sugar manufacturing process include sugarcane trash, sugarcane bagasse (SCB), press mud cake (PMC) and SCB fly ash ( Fig. 1 ). The key characteristics of these solid wastes are summarized in Table 1 . Although cogeneration for steam and electricity production is the most harnessed industrial route for SCB valorisation, 2 high value co-product and biofuel production from SCB and other wastes has also been explored. 3 For example, SCB is also used as a raw material in agro-residue based pulp and paper mills. Other SCB based products with added value are furfural and animal feed. Another example is PMC that is rich in organic content, phosphate, calcium and magnesium and therefore is used as a fertilizer after bio-composting with biomethanated spent wash (distillery wastewater). Attempts have been made to utilise PMC as a fuel in sugar mills either alone or in combination with SCB; however it leads to the generation of 25% more ash than SCB. 4 Sugarcane trash is conventionally disposed by burning in the fields. SCB fly ash is typically disposed in pits; however it is also applied on land for soil amendment in some areas or used for brick manufacturing. In addition to the solid wastes generated during sugar production, a significant portion of mother liquor left behind after crystallisation of sugars from juice ends up as a liquid waste – molasses (4% of sugarcane crushed). Molasses is typically used to produce ethanol via fermentation. Many sugar mills also run distilleries as their subsidiaries for producing fuel ethanol and extra neutral alcohol to maximise profit margins. The additional profits become critical especially because, in the recent years, due to the excess production of sugarcane, the sugar inventory has been piling up in India leading to a crisis. In addition, due to decreasing international prices of sugar, paying a fair and remunerative price (FRP) to the farmers has also become a challenge. In view of the sugar crises, the Government of India (GoI) took a policy decision to implement the ethanol blending programme (EBP) and to go for 10% blending (in petrol) throughout the country and cut oil imports (20% by 2030). Furthermore, to increase profits with molasses based ethanol, efficient waste management is required to valorise the waste streams into value added products.
Amongst the waste generated by the sugar industry, SCB contributes to a significant proportion (∼100 million tonnes per annum). It is also one of the largest agriculture residues in the world. 5–7 This waste does not just present a challenge, but also creates an opportunity where proper waste resource management can lead to additional revenue. This revenue will help in tackling the problems faced by the Indian sugar industry such as the falling sugar prices, surplus sugar production and payment of FRP. A preliminary review of the literature indicates that SCB can serve as a potential feedstock for low cost production of green chemicals and fuels. This is mainly attributed to its abundant availability making its supply constant and stable.
The current SCB utilization approach in India is restricted to cogeneration (of steam & power). Considering the valorisation potential of SCB, cogeneration is not the best option (although mature). There is limitation on use of SCB for cogeneration due to the diminishing market price of electricity. There will be a high demand for ethanol [>9 billion litres by 2030 ( ref. 8 )] with excellent differential pricing offered by the GoI based on use of different intermediates/by-products of sugarcane processing. In future, the economics of the sugar industry will not only depend on sugar, ethanol or cogeneration but will also depend on the optimal use of (wastes) resources available within the sugar industry. Meghana and Shastri's recent review on the global sugar industry with a strong focus on environmental impacts suggested that monetising the environmental impacts will also act as a driver towards utilising these wastes as resources 2. This paradigm shift to waste-based biorefineries with a circular economy approach is therefore the way forward for the Indian sugar industries.
With this background, this review aims to examine innovative value-added products in addition to fuels that can be obtained from the transformation of SCB in an Indian context. Such initiatives towards a biorefinery are expected to promote alternative approaches to steam and power. This work presents the state-of-the-art in this field especially focusing on high value products. The manuscript is structured in a way to first describe the potential of SCB as a feedstock for biorefineries, followed by the importance of pre-treatment in enhancing product yields. Finally, routes and processes for gaseous and liquid products, reported yields and challenges in adopting these SCB valorisation solutions in a biorefinery are highlighted.
Sugarcane bagasse (SCB) and its potential as a feedstock for biorefineries
In the crushing season 2018–19, sugarcane crushing, sugar and SCB production was 303.83 million tonnes, 32.82 million tonnes and 91.15 million tonnes in India respectively. 1 SCB cogeneration in India picked up pace after the ‘National programme on promotion of biomass power/bagasse-based cogeneration’ was implemented by the GoI in 1992. As it leads to environmental and economic sustainability, the GoI formulated many promotional policies for setting up more combined heat and power (CHP) plants, which further encouraged such sugar plants to adopt cogeneration technology. 17 During the last two decades, many sugar mills have installed co-generation (power/steam) plants from 10 MW h −1 to above 50 MW h −1 capacities. It helped in earning additional revenue and allowed sugar mills to pay a higher cane price to farmers. The current total installed capacity of these cogeneration plants in India is 9200 MW. Despite its use in boilers, a significant amount of SCB still remains as waste. As mentioned previously, the SCB based cogeneration electricity prices are going down, as cheap electricity is now available from other sources. Therefore, there is a need to identify other valorisation routes that can help in effective SCB management with a surplus economic gain.
In the present scenario, the production of chemicals is completely reliant on fossil fuels which is non-sustainable and has a negative impact on the environment. As a result, there is a growing demand for clean, greener and sustainable technologies to manufacture fuels and chemicals that requires a paradigm shift from petrochemical based synthesis towards bio-based production. 27 The main bottleneck in the commercial success of biorefineries is high cost which stems from feedstocks and their pre-treatment. Profitable biorefineries could be realized by making use of waste streams rich in renewable carbon and substantially reduce the production cost and spare edible feedstocks. 28 As mentioned earlier, India being the second largest producer of sugarcane crop in the world generates massive amounts of SCB as waste. SCB is an inexpensive source of fermentable sugars and a potential feedstock for second-generation biorefineries. It provides a significant opportunity for biofuel/biochemical/chemical industries in India and has potential for rural economy development. Being a lignocellulosic feedstock with a low nutritional value, the use of SCB precludes concerns about the food vs. fuel debate, especially in a country like India with a huge population. The current use of SCB for power generation or CHP is a low grade application and does not utilize its full potential. The annexation of second generation (2 G) biorefineries into existing sugar mills through more efficient use of waste streams such as SCB will lead to revitalisation and sustainability of sugar industries. 28,29
In 2017, the GoI announced a call, in association with UK, on the “Industrial Waste Challenge” to find green and sustainable solutions for waste streams generated by five major industries in India and sugar mills were one of them. Our consortium (vWa) was funded under this call and we proposed valorisation of SCB to five products of huge commercial value: bioCNG, xylitol, n-butanol, lactic and succinic acid and are working towards it. Potential products from SCB and their theoretical yields are listed Table 3 . The key challenges in the valorisation of SCB via a biochemical route are: development of a cost-effective pre-treatment method to make the biomass amenable to further biotransformation, simple & cost effective detoxification, effective hydrolysis (without or in house low cost enzymes) and biotransformation of sugar in hydrolysates to the desired products which meet customer specifications without compromising the safety, environment and economics.
Pre-treatment of sugarcane bagasse
Pre-treatment methods are generally classified into four categories; physical, chemical, biological and physico-chemical pre-treatment ( Fig. 2 ). Comminution is the most common physical pre-treatment where SCB is milled to a desirable particle size range. Milling not only helps in reducing the particle size or increasing the specific surface area, but concomitantly also affects the cellulose crystallinity. The combined effect of an increased surface area and reduced crystallinity in turn facilitates enhanced enzymatic hydrolysis thereby improving the saccharification rate and yield. This in turn facilitates enhanced enzyme binding to the cellulose fractions of the biomass thereby improving the saccharification rate and yield. 31,32 Milling performance and the specific milling energy required are dictated by parameters such as the moisture content of SCB, required comminution ratio (ratio of initial particle size to final particle size) and the type of mill used. An increased moisture content leads to an increased energy draw and plugs the meshes. An increase in the comminution ratio (decrease in the final particle size) also leads to an increase in the specific milling energy. For instance, using a bench scale knife mill, Miao et al. reported that ∼720 kW h per tonne dry weight is required to grind SCB (∼40% moisture content) from an initial size of 20–25.4 mm to a final particle size of 2 mm. 33 When the final particle size was 8 mm, the energy requirement reduced to ∼390 kW h per tonne dry weight. The aforementioned factors affect dry milling, whereas an alternative wet milling approach has also been exploited in the literature to pre-treat SCB. For instance, da Silva et al. reported the use of wet disk milling as a method to pre-treat SCB for enhancing the saccharification yield. 31 Lab scale milling pre-treatment for enhanced saccharification of SCB is often reported; 34,35 however, the most important parameter, the specific milling energy requirement, is hardly reported. In any case, the energy consumption values based on lab scale milling will not be relevant for industrial scale milling. The data on energy requirements for large scale milling are not readily available.
Chemical pre-treatment typically utilises an acid or an alkali to hydrolyse or delignify the biomass. They may be used in conjunction with compressed hot water or saturated steam, so that the chemical pre-treatment method becomes a physico-chemical method. Chemical pre-treatment methods often end up producing inhibitors (of fermentation) such as 5-hydroxymethylfurfural, acetic acid, formic acid or furfural, under acidic conditions. The hydrolysates when not detoxified appropriately will negatively influence the subsequent fermentation. Furthermore, as a general rule, biomass slurries upon chemical pre-treatment need to be neutralised prior to fermentation/biogas generation and therefore may incur additional costs at scale.
Unlike chemical pre-treatment, biological pre-treatment involves the use of enzymes or microbes to delignify or hydrolyse SCB. A class of fungi known as the brown rot fungi produces extracellular lignolytic enzymes such as lignin peroxidases or laccases that help in delignification. A close ally, white rot fungi on the other hand can produce lignocellulolytic enzymes that can also hydrolyse cellulose (cellulase), in addition to breaking down lignin. 36 While biological methods have proven to be advantageous for delignification with low input energy requirements, the slow rate of pre-treatment is often seen as a significant disadvantage. On the other hand, the process also becomes expensive when highly pure enzymes are utilised for targeted pre-treatment and fractionation.
However, it may be possible to recover and recycle enzymes to reduce the overall pre-treatment cost. 47
Physico-chemical methods are attractive pre-treatment options as they potentially overcome the disadvantages posed by other techniques. Steam explosion with a mild acid or alkali has particularly gained interest for SCB pre-treatment to enhance its saccharification potential and in turn bioethanol yields. 46,48–50 Furthermore, it has also been proposed to be an energy efficient pre-treatment method for bioethanol production. 51 The rapid depressurisation during steam explosion facilitates physical pre-treatment by breaking the fibres while the acid/alkali will catalyse hydrolysis or delignification of the biomass. The process conditions can be tuned to obtain C5 sugar rich hydrolysate and cellulose rich residue. Physico-chemical pre-treatment seems a promising method in terms of scalability and energy efficiency, not only for saccharification or bioethanol production but also for enhancing biogas yields via anaerobic digestion (AD) of SCB. For instance, the use of hydrodynamic cavitation (HC) as a biomass pre-treatment method is increasing rapidly. 41,52,53 HC is the phenomenon of formation, growth and implosion of vaporous cavities. HC occurs in a flowing fluid as a result of a local drop in pressure due to the design geometry. During HC pre-treatment, reactive radical species generated in situ from the cleavage of water molecules chemically pre-treats the biomass, whereas the collapsing cavities induce shear and physically pre-treat the biomass, affecting the particle size and morphology.
Various pre-treatment categories were introduced earlier in the section; however, it is important to match specific methods to the end applications. For example, anaerobic digestion being a robust technology can handle toxins well when compared to classic sugar fermentation; therefore, detoxification maybe required prior to fermentation, whereas they may not be necessary for biogas generation applications. Similarly, breaking open the biomass structure would be sufficient to enhance biogas yields, whereas fractionation is required for enhanced saccharification and fermentation. Therefore, a few examples from the literature are specifically presented for these two main applications, namely (i) biogas production ( Table 4a ) and (ii) saccharification (both C5 and C6 sugars) leading to fermentation ( Table 4b ).
The simplest pre-treatment that can be performed for enhancing biogas generation is comminution. K. Kivaisi et al. reported an enhanced biogas generation of up to 33% for a comminution ratio of 5.8 with the final SCB particle size being 0.85 mm. 37 A. F. Leite et al. on the other hand reported an enhancement of up to 15% in biogas yield for a comminution ratio of 10 for a final particle size of 1 mm. 38 Although the final particle sizes were similar in both cases, with the comminution ratio for Leite et al. being almost twice as much as the former, the improvement in biogas observed was almost half as much. While both the groups used SCB that had a VS content of over 90% (on dry basis), the lignin content differed significantly (Kivaisi & Eliapenda reported a lignin content of SCB 9% as compared to Leite et al. at ∼16%), which may have influenced the variation in the enhancement observed.
Chemical methods have been reported extensively to improve biogas generation. For instance, Rabelo et al. (2011) utilised calcium hydroxide (lime) to pre-treat SCB at 90 °C for 90 h. 39 They used a lime loading of 0.47 g g −1 SCB and observed a decrease in lignin and hemicellulose content from 23% to 20% and 25% to 13%, respectively. A corresponding increase in the cellulose content in the solid residue up to 66% (from 38%) was also reported. When alkaline hydrogen peroxide treatment was opted (7.36% v/v) at room temperature for 1 h to pre-treat SCB, a much higher increase in the cellulose content was observed in the residue (81%). Both these methods favoured enhanced biogas yields from the solid residue upon pre-treatment. Additionally, to test the possibility of a biorefinery, the solid residue rich in cellulose was utilised for saccharification and the liquor was subjected to biomethanation potential (BMP) tests. Alkaline peroxide treatment was favourable for supporting biogas generation from the liquor due to the higher quantity of hemicellulose solubilised from SCB (corresponding xylan content in solids reduced from 25% to 8%). Costa et al. (2014) utilised conventional sodium hydroxide pre-treatment to delignify SCB (1 mm particle size). 40 They reported a 26% lignin removal that resulted in a 3.8 fold increase in biomethane production to 139 L CH 4 per kg substrate. While alkali pre-treatment is generally favoured for delignification, acid hydrolysis is exploited for solubilising hemicellulose (minimal acid soluble lignin) to produce cellulignin (solid residue rich in cellulose and lignin). However, the type of acid influences the outcome upon pre-treatment. For example, for enhancing biogas production, when H 2 SO 4 is used to pre-treat SCB, the sulfates formed during the neutralisation stage have to be removed; otherwise, the biomethane yields will be compromised. This is due to the action of a class of bacteria known as the sulfate reducing bacteria (SRB) present in the digester. SRB utilises sulfate and compete with methanogens for protons from the feedstock/volatile fatty acids, thereby reducing the available protons for methane generation. Costa et al. therefore reported the use of HCl for the pre-treatment of 1 mm SCB particles for enhancing the biomethane production. They achieved >50% solubilisation of holocellulose that favoured an increase in BMP up to 122 L CH 4 per kg substrate (3.4 times increase). The increase in BMP upon acid pre-treatment was lower as compared to alkali pre-treatment, reiterating the fact that lignin induced recalcitrance hinders holocellulose bioavailability and therefore must be removed.
Nagarajan and Ranade (2019) reported a novel vortex based HC pre-treatment to enhance the biogas yields from SCB. The novel vortex based device used by S. Nagarajan et al. 41 harnessed HC using a vortex flow based device invented by. 54 It has eminent advantages over conventional linear flow devices, such as no small constrictions and cavitation occurring in the core and away from the walls leading to no device erosion and hence requiring minimum maintenance. Additionally, the energy required for vortex based HC pre-treatment of SCB was reported to be 140 kW h per tonne dry weight (for a low biomass loading of 1%), which was significantly lower than the milling energy mentioned earlier and other HC devices used for agricultural residue pre-treatment. 55,56
Mechanical grinding is known to improve the enzymatic saccharification efficiency. Accordingly, milling pre-treatment for enhanced saccharification of SCB is often reported. 31,34,35 da Silva et al. compared ball milling and wet disk milling of 2 mm SCB particles based on the saccharification efficiency. With the untreated SCB yielding 22% glucan upon enzymatic hydrolysis, ball milled SCB yielded >80% and wet disk milled SCB yielded 49%. The ability of ball milling to completely convert the crystalline cellulose in SCB to amorphous cellulose was attributed as the reason for such a high saccharification yield.
Deswal et al. (2014) utilised three white rot fungi, namely, Pleurotus florida, Coriolopsis caperata RCK 2011 and Ganoderma sp. rckk-02, to delignify SCB. 43 The highest delignification of 8% was observed when P. florida was used to treat SCB for 25 days. The solid residue upon recovery was subjected to enzymatic saccharification that yielded 49% reducing sugars as compared to untreated SCB (20%). More recently, Machado and Ferraz reported delignification of SCB using Ceriporiopsis subvermispora . 44 The autoclaved material when subjected to fungal fermentation facilitated the removal of 47% lignin and 48% hemicellulose in 60 days. This favoured the enzymatic saccharification of cellulose rich residue and increased the glucan yield to 55% from 23%.
Carrasco et al. (2010) performed steam explosion of SO 2 impregnated SCB under their optimised conditions of 190 °C for 5 min to obtain a xylose yield of ∼60%. 45 Fermentation inhibitors such as acetic acid, furfural and 5-hydroxymethylfurfural were also observed in the hydrolysate along with xylose and xylo-oligomers. The solid residue upon pre-treatment was saccharified using an enzyme cocktail that yielded 90% glucose at 2% solid loading. Carvalho et al. (2018) were able to achieve similar xylose yields by using a higher concentration of SO 2 in combination with a lower temperature (3%, 150 °C and 30 min). 48 Swapping SO 2 with 0.5% H 2 SO 4 and reducing the time by half also yielded similar concentrations of xylose in the hydrolysate. They also demonstrated that autocatalytic steam explosion (without an added catalyst) is selective towards xylo-oligosaccharides whereas in the presence of an acid catalyst, xylose production was favoured. More recently, autocatalytic steam explosion at 190 °C for 15 min was performed with 80 g L −1 SCB in a 250 L pressure reactor by G. J. M. Rocha et al. 46 to recover hemicellulose rich hydrolysate (∼90%). The solid residue (cellulignin) was further treated with 1% NaOH aqueous solution under oxygen pressure to solubilise lignin and leave behind a pure cellulose rich solid residue. With tuneable parameters paving way for selective fractionation and inhibitor minimisation, steam explosion is a suitable candidate for pilot and industrial trials aimed at bioethanol production. Accordingly, there have been a few studies at the demo scale level to produce xylose rich hydrolysates continuously from SCB ( e.g. DBT-ICT technology and Praj Industries, India). 57–59
Other pre-treatment methods have also been utilised for SCB pre-treatment such as microwave, 42 compressed hot water, 60 organosolv, 61 ionic liquids, 62 ammonia fibre explosion, 63 supercritical CO 2 , 64 acoustic cavitation, 65 photocatalysis, 66 gamma radiation, 67 Fenton's reagent 68 and ensiling pre-treatment. 69 These methods are however not discussed in this review as they are mainly restricted to the lab scale, expensive, energy intensive or not scalable at this point of time. The readers are henceforth directed to the references directly to know more on these methods. Amongst the pre-treatment methods discussed, (catalytic) steam explosion and hydrodynamic cavitation for bioethanol production and biogas generation respectively seem promising options for scale up with economic feasibility and therefore may be used to realise a bagasse based biorefinery.
SCB to biogas
Neither AD nor biogas is alien to India. In fact, the world's first biogas plant was built in Bombay (now Mumbai), India in 1859 that utilised sewage sludge as the feedstock. 70 Currently, the total biogas production in India is 2.07 billion m 3 per year. This is quite low compared to its potential, which is estimated to be in the range of 29–48 billion m 3 per year. 71 In the current context, with the policy drivers in India clearly pointing towards a biogas market, it is important that the current subsidies provided by the GoI are leveraged. To build base and boost the capacity for biogas generation, the Ministry of Petroleum and Natural Gas initiated the Sustainable Alternative Toward Affordable Transportation (SATAT) scheme in late 2018. 72 The crux of this scheme was to create a buy in for CBG (compressed biogas) produced from waste biomass sources (₹46 per kg CBG) 73 and utilise it as a sustainable transportation fuel. The initial expression of interest call aimed at supporting the phased setup of 5000 biogas plants spanning across India. Recently, the Reserve Bank of India (RBI) has also included CBG plants under priority sector lending. With India being the world's second largest producer of sugarcane (303.83 million tonnes in 2019) and ∼30% ending up as SCB (91.15 million tonnes) and operating over 500 sugar mills, the availability of SCB as a feedstock for biogas generation via AD fits the purpose precisely. As per the notification from the Department of Agriculture and Farmers Welfare, GoI (13 th July 2020), the fermented organic digestate coming out from AD of SCB/other agro-wastes is now approved as organic manure in Fertilizer Control Order (FCO). The enhanced and readily available nitrogen in the digestate when used as an organic fertilizer would partly or fully offset the need for chemical fertilizers which in itself has a high energy demand during production. 74 This will immensely help the biogas and CBG sector in future.
Biogas generation from SCB is dependent on its composition, particle size and morphology. The cellulose and hemicellulose fractions together in SCB constitute about 60–70% of its dry weight (or total solids, TS) and can be predominantly utilised towards biogas generation. Lignin generally remains unaffected; however it can hinder the effective utilisation of polysaccharides. Therefore, complete conversion of the holocellulose fraction is unlikely. Inherent variability in the composition of SCB can occur due to the species of cane utilised, time of harvest or varying efficiencies of juice extraction. For instance, Janke et al. (2015) sampled SCB from plants located in two different Brazilian states in two different seasons and tested the biochemical methanation potential (BMP) of these variants. With the volatile solids (VS) content not overly different (96 ± 2.7% of TS), a huge variation in BMP was observed between the two samples, namely 236 and 326 mL CH 4 per g VS. They attributed this difference to the varying quantities of easily utilisable residual non-structural carbohydrates in SCB present upon juice extraction. Using the elemental composition of SCB, theoretical BMP can be calculated using the Buswell equation. The theoretical maximum varies in the range of 425–487 mL CH 4 per g VS for SCB. 41 Theoretical BMP is calculated based on the assumption that all VS can be utilised towards biogas generation. In reality, however this can never be achieved. The experimental BMP of SCB reported in the literature spans over a broad range from 37 (assuming a VS content of 96% of TS) 40 to 326 mL CH 4 per g VS, 75 while the typical range is 170–250 mL CH 4 per g VS. Pre-treatment has the capability to improve the BMP. The influence of pre-treatment on biogas production with typically observed biogas generation profiles is captured in Fig. 3 . A variety of studies reporting an increase in BMP upon SCB pre-treatment are summarised in Table 5 .
Sajad Hashemi et al. (2019) subjected milled SCB (1 mm) to ethanolic ammonia pre-treatment to enhance the BMP. 76 An equal weight of 10% v/v aqueous ammonia was mixed with a range of ethanol–water mixtures (5–50%) and 10 g milled SCB was soaked to the resulting ethanol–ammonia aqueous solution. Pre-treatment was performed in a closed reagent bottle at either 50 or 70 °C for 12 or 24 h. The BMP of the untreated milled SCB, neutralised solid fraction, ethanol-ammonia free liquid and slurry were utilised for batch mesophilic BMP tests. The highest BMP was obtained from the solid (249 mL CH 4 per g VS) and liquid (298 mL CH 4 per g VS) fractions (pre-treated at 70 °C for 12 h with 10% ammonia and 25% ethanol). In the case of the slurry, an increase in BMP was observed with an increase in pre-treatment severity (299.3 mL CH 4 per g VS upon pre-treatment at 70 °C for 24 h with 10% ammonia and 50% ethanol). The obtained methane yield was over 2-fold higher than that observed with untreated milled SCB. The delignification and breakdown of lignin-carbohydrate bonds were ascribed to be the reasons contributing towards an enhanced BMP.
Leite et al. (2015) performed batch BMP experiments with raw SCB (∼10 mm particle size), milled SCB (1 mm) and lime treated milled SCB (10% w/w, lime/SCB in 24 mL water for 24 h). 38 They achieved a 20% increase in biomethane yield upon milling pre-treatment, whereas an alkali pre-treatment step post milling improved the yield by 50%. They suggested that lime treatment might be economically feasible upon recycling the liquid phase; however, no cost analysis was presented. Mustafa et al. (2018) also utilised lime pre-treatment as a strategy to enhance the BMP of 3 mm SCB. 77 They used 8.5% lime and pre-treated SCB for 4 days to achieve an increase in biogas yield by 23%. They attributed the enhancement to the delignification observed as a result of lime pre-treatment. When hydrothermal pre-treatment (compressed hot water) was opted at 180 °C for 20 min, a >35% increase in biogas yield was observed. The methane content in biogas for all the cases was observed to be over 65%. Upon hydrothermal pre-treatment, a diauxic digestion trend was observed which indicates that there may have been production of inhibitors during pre-treatment. When hydrothermal pre-treatment was combined with lime pre-treatment sequentially, the biomethane yield enhancement observed was over 61%. In contrast to hydrothermal pre-treatment, a single digestion stage was observed upon sequential pre-treatment indicating that the inhibitors that might have been produced did not interfere with the microbial metabolism. The enhanced energy that was produced upon sequential pre-treatment was calculated to be 366 kW h per tonne dry weight (difference of energy in methane obtained from pre-treated SCB and untreated SCB). Without taking into account the energy required for milling, considering the energy required to heat water for hydrothermal pre-treatment (210 kW h per tonne dry weight), the sequential pre-treatment yielded biomethane with a net energy gain of 156 kW h per tonne dry weight.
Nagarajan and Ranade (2019) used a vortex based HC device to pre-treat 2 mm SCB particles at a solid loading of 1% (w/w) for 9 passes through the device. 41 The energy required for pre-treatment was 140 kW h per tonne dry weight (for 1% solid loading) and the net energy gain achieved was 398 kW h per tonne dry weight from the enhanced biomethane yield achieved (24%). The modified surface morphology, particle size reduction and chemical compositional alternation due to cavitation pre-treatment were attributed to be the reasons for an enhanced biomethane production. Assuming that the energy gain due to the enhanced biomethane generation stayed constant at 537 kW h per tonne dry weight for various solid loading, the net energy gain will increase with increased solid loading. For example, 5% solid loading (based on the dry weight of SCB) will enhance the net energy gain to more than 500 kW h per tonne of dry SCB with 9 pass HC pre-treatment. HC therefore appears to be a promising method to pre-treat SCB for enhancing the biomethane production. Additionally, hybrid treatment methods such as alkali in combination with HC may also be tested to specifically improve the delignification of SCB for further enhancing the BMP.
AD when compared to other renewable energy technologies requires the least capital cost. 83 To further add value, AD should not only be considered for biogas generation, but also be considered as the base of a viable biorefinery. SCB may be partially converted in AD and the resulting digestate would contain the unconverted holocellulose fraction with a loosened fibre matrix due to microbial activity. 84 This digestate may be used for further value addition without needing expensive chemical or enzymatic pre-treatment. Thus, AD may be used as a base for the biorefinery not only to recover energy in the form of biogas/CBG, but also to open up the lignocellulosic matrix of unconverted biomass in the digestate which can be converted to sugars and other value added products (see the next section).
SCB to alcohols and acids
Neves et al. (2016) investigated cellulosic ethanol production from native as well as ethanol extracted SCB. 49 The extraction with ethanol was performed to reduce the total extractive content for the removal of inhibitory compounds. They made use of the steam explosion method with three different configurations for the pre-treatment of native and ethanol extracted SCB: autohydrolysis and dilute acid hydrolysis with H 2 SO 4 and H 3 PO 4 . The pre-treated SCB was subjected to enzymatic hydrolysis for glucose release using commercial cellulase preparation Cellic CTec2 followed by fermentation with an industrial strain of S. cerevisiae . Similar results were obtained for glucose recovery and ethanol production with three pre-treatment methods. The ethanol production in terms of titer and yield was better with SHF than with SSF. The highest ethanol titer (27.1 g L −1 ) and yield (0.47 g g −1 ) were obtained during SHF using SCB pre-treated by autohydrolysis. On the other hand, the total reaction time was lower in SSF (48 h) than in SHF (84 h) leading to high volumetric productivities. The maximum ethanol productivity was achieved (0.58 g L −1 h −1 ) when SSF was carried out after pre-hydrolysis with a total processing time of 24 h. Though ethanol extraction removed 80% of organic solvent extractable materials from SCB, this extraction had no impact on ethanol production regardless of the pre-treatment method and fermentation strategy. In a recent study, de Araujo Guilherme et al. (2019) employed different strategies to optimize the ethanol production from SCB through SSF in batch and fed-batch modes. 90 They made use of four pre-treatment methods (acid, alkali, hydrothermal and hydrogen peroxide) and four microbial strains for ethanol production. In addition, the enzyme dose and inoculum size were optimized. The highest cellulose content of 65% was achieved with acid-alkali pre-treatment and was most favourable towards ethanol production. The synergistic effect of two pre-treatment favoured the removal of lignin and hemicellulose by acid and alkaline steps, respectively. Furthermore, the combined pre-treatment resulted in the reduction of crystallinity of cellulose. Among the four strains used, the maximum ethanol yield was obtained using S. cerevisiae PE-2 and cellulosic carbon after acid-alkali pre-treatment. The batch SSF process using optimized parameters (inoculum size: 1 g L −1 , enzyme dose: 15 FPU cellulase per g cellulose and initial cellulose: 6%) resulted in an ethanol titer, yield and productivity of 31.5 g L −1 , 0.47 g g −1 and 1.75 g L −1 h −1 . The fed-batch cultivation with three times less enzyme dose in comparison to the batch process yielded 29.8 g L −1 in 40 h with 0.45 g g −1 yield. To overcome the limitations of SSF, Liu et al. (2016) included pre-hydrolysis of alkali pre-treated SCB, i.e. after 24 h of pre-hydrolysis at 50 °C, the temperature was reduced to 37 °C and inoculated with yeast for SSF. 91 With this approach, they were able to enhance the solid loading up to 36%. Ethanol fermentation was conducted in batch and fed-batch modes with pre-hydrolysis SSF. The maximum ethanol titer of 66.9 g L −1 was achieved with a conversion efficiency of 72.9% in 96 h in fed-batch mode with 30% (w/v) loading. Despite much efforts, the use of SCB as the feedstock for ethanol synthesis is still far from commercialisation due to expensive pre-treatments, moderate titers and low volumetric productivities. 92,93
To incentivize the 2G ethanol sector and support and create a suitable ecosystem for setting up commercial projects and increasing Research & Development, the Government of India on 28-Feb-2019 launched the “Pradhan Mantri JI-VAN (Jaiv Indhan Vatavaran Anukool Fasal Awashesh Nivaran) Yojana” as a tool to create 2G ethanol capacity in the country and attract investments in this new sector. The said scheme has been notified on 08-Mar-2019 in the Extraordinary Gazette of India. The scheme objective is to support 12 Commercial Scale and 10 demonstration scale 2G ethanol projects with a viability gap funding with a total financial outlay of ₹1969.50 crores for the period 2018–19 to 2023–24. Out of the ₹1969.50 crores, ₹1800 crores have been allocated for supporting 12 commercial projects, ₹150 crores have been allocated for supporting 10 demonstration projects and the remaining ₹19.50 crores will be provided to the Centre for High Technology (CHT) as administrative charges.
Our consortium worked towards xylitol production using SCB from Indian sugar mills. The xylose rich hemicellulosic hydrolysate for this purpose was provided by our industrial partner Nova Pangaea Technologies. We used two different yeast strains with the GRAS status for the biotransformation of xylose into xylitol: Yarrowia lipolytica and Pichia fermentans . Y. lipolytica is a non-conventional oleaginous yeast lacking the ability to grow on xylose; however, the cell mass of yeast can transform xylose into xylitol. The high cell density of yeast was accumulated on glucose/pure glycerol/crude glycerol followed by the transformation of xylose into xylitol and the conversion yields obtained were ≥90%. When pure xylose was replaced with hemicellulosic hydrolysate in an optimized medium, the yield dropped to 54% ( ref. 97 ) ( Fig. 4 ). The observed drop in yield might have been due to the inhibitors in hydrolysate which would have been overcome with suitable detoxification. Although this preliminary work was positive, more work is required to achieve superior performance. In another study 98 (manuscript under review), a xylose eating and xylitol accumulating yeast P. fermentans was isolated. The wild type strain was subjected to random mutagenesis and a mutant strain outperforming the wild type was identified. The culture medium was optimized using a statistical method and the process was scaled up from a shake flask (250 mL with a working volume of 50 mL) to a bioreactor level (2.5 L with a working volume of 1.0 L). After media optimization, the mutant strain produced a maximum xylitol titer and yield of 70.5 g L −1 and 0.49 g g −1 respectively from pure xylose. While on xylose rich hydrolysate, the strain was able to accumulate 62.3 g L −1 xylitol with a conversion yield of 0.43 g g −1 . Upon scaling up using an optimized media composition, the xylitol titer and yield improved to 98.9 g L −1 and 0.67 g g −1 , respectively with pure xylose. In the case of hydrolysate, the xylitol concentration and conversion yield enhanced to 79 g L −1 and 0.54 g g −1 respectively ( Fig. 5 ).
The integrated biorefineries for simultaneous synthesis of ethanol and xylitol could enhance the profitability of cellulosic ethanol production by 2.3-fold. 94 Therefore, the co-production of ethanol and xylitol from cellulosic and hemicellulosic fractions has been attempted to improve the process economics of SCB-based biorefineries. Xylitol has a higher market value than ethanol; therefore, an integrated SCB processing for co-production would create economic benefits making the overall process feasible. 99 Two different approaches have been employed for the co-production: use of a single organism for two products, one strain for each metabolite. da Silva et al. (2015) and Dasgupta et al. (2017) used Kluyveromyces marxianus for co-production. 95,100 K. marxianus produced ethanol (titer: 12 g L −1 ; yield: 0.22 g g −1 ; productivity: 0.08 g L −1 h −1 ) from cellulosic hydrolysate. While with hemicellulosic hydrolysate, xylitol (titer: 9.4 g L −1 ; yield: 0.40 g g −1 ; productivity: 0.10 g L −1 h −1 ) was obtained as the main product with ethanol (1.31 g L −1 ) as a by-product (da Silva et al. 2015). In a second approach, Castañón-Rodríguez et al. (2015) utilised two different yeasts, S. cerevisiae and C. tropicalis which are best known for their ability to ferment glucose and xylose to ethanol and xylitol, respectively. 101 These two yeast strains were co-cultured using a simulated medium of SCB hydrolysate instead of separate fermentation for ethanol and xylitol. The best condition for co-culture was sequential addition where fermentation was initiated with S. cerevisiae and 24 h later, C. tropicalis was inoculated. As a result, the glucose concentration reduced to a low level to allow efficient utilization of xylose. The fed-batch cultivation with sequential co-culture resulted in enhanced production of both metabolites, ethanol (titer: 11.6 g L −1 ; yield: 0.44 g g −1 ; productivity: 0.87 g L −1 h −1 ) and xylitol (titer: 8.5 g L −1 ; yield: 0.57 g g −1 ; productivity: 0.27 g L −1 h −1 ). In another study by Unrean and Ketsub, 2018, S. cerevisiae and C. tropicalis were cultured separately for the production of ethanol and xylitol. The fed-batch cultivation resulted in the highest concentrations of ethanol by S. cerevisiae and xylitol by C. tropicalis at 56.1 g L −1 and 24 g L −1 with product yields of 0.44 and 0.50 g g −1 respectively.
Significant research has been done in the last few decades on bioproduction of xylitol and substantial improvement in the titer, yield and productivity of xylitol has been achieved using metabolic and process engineering approaches. Despite all this, the bioprocess is still far from industrial level production. Even today, the chemical route for xylitol production remains the dominant one. 94,96 According to a simulation study by Mountraki et al. (2017), the amount of xylitol crystals which can be obtained from 1 kg of xylose via chemical and biotechnological routes was 0.87 and 0.73 kg, respectively. 102 In order to make the biological route competitive to its chemical counterpart, several hurdles need to be overcome to cut down the high capital and operational cost of various steps such as pre-treatment, detoxification, fermentation and downstream processing. Table 6 summarises the xylitol production from SCB by different microorganisms and the comparison reveals that our results outperformed other previous reports, making it competitive. Most of the studies in Table 6 have detoxified the hydrolysate using methods such as over-liming, activated charcoal, ion exchange resin adsorption treatment or a combination of these methods for improving fermentation performance. Detoxification adds to the operational costs and may also lead to loss of sugars. Thus, a cost effective and convenient pre-treatment with minimum or no release of inhibitors eliminating the detoxification step along with efficient recovery of xylitol is highly desirable. This in combination with the design of a hyper and robust xylitol producer with the aid of advanced metabolic engineering and synthetic biology, process design, intensification and integration with sugar mills can enhance the commercial feasibility of xylitol production at the bulk level.
Succinic acid
The batch fermentation of non-detoxified hemicellulosic hydrolysate containing 22.5 g L −1 xylose, 3.6 g L −1 glucose and 3.9 g L −1 arabinose was performed in a bioreactor. All the sugars were consumed, and SA achieved at the end of fermentation was 23.7 g L −1 . The yield and productivity were 0.79 g g −1 and 0.99 g L −1 h −1 . The hydrolysate contained 2.84 g L −1 total soluble phenolic compounds, 0.42 g L −1 HMF, and 0.71 g L −1 furfural. Despite this, surprisingly, the results obtained with non-detoxified hydrolysate were better than those of the detoxified one. The SA titer and yield achieved were 20.9 and 20.2% higher in comparison to detoxified hydrolysate showing that detoxification was not required. No specific explanation was offered.
Bacteria are extremely sensitive towards low pH and require moderate pH for their growth, resulting in the consumption of large quantities of the neutralizing agent that affects the productivity cost. 117 Further at neutral pH, SA is obtained in the form of succinate salts and needs an additional step of acidification to bring it back to the acid form leading to accumulation of by-products such as gypsum, in-turn making the downstream process more critical. This problem can be resolved by carrying out the fermentation at a low pH value. 118 Obtaining SA in an unionized form will simplify the downstream processing and lead to an economical process as it will avoid neutralization during fermentation and acidification during product recovery. 104 Yeasts are potential host to produce organic acid because of their high tolerance to low pH and are naturally predisposed to grow under low pH, below 4. 119 Yarrowia lipolytica is an oleaginous and non-conventional yeast and excellent cell factory for synthesizing a large variety of commercially important products. 120 It has an amazing ability to grow perfectly well over a wide pH range without significant change in growth parameters. 121 In a recent report by Ong et al. (2019), the feasibility of SA production from co-fermentation of glucose and xylose by Y. lipolytica was investigated. 122 They utilised pure as well as crude glucose and xylose from SCB as the feedstock. To maximize sugar release from pre-treated SCB, enzymatic hydrolysis was optimized through three parameters: pH, temperature and enzyme dosage. They reported 33.2 g L −1 SA by Y. lipolytica using SCB hydrolysate containing 47.3 g L −1 glucose and 20.2 g L −1 xylose during bioreactor cultivation. Glucose was completely consumed by the yeast; however, a large fraction of xylose remained unutilized (50–70%). Our consortium also produced SA from SCB hemicellulosic hydrolysate by Y. lipolytica without the control of pH. 123 The yeast can utilize a variety of carbon sources but lacks the ability to metabolize xylose. Y. lipolytica was engineered for xylose assimilation and SA production. The batch cultivation of recombinant strain in the bioreactor resulted in a SA titer of 11.2 g L −1 with a yield of 0.18 g g −1 . The experiment was repeated with crude xylose (40 g L −1 ) rich hydrolysate derived from SCB. Hydrolysate after pre-treatment often contains inhibitors which can negatively impact the performance of microorganisms. The cell growth was unaffected as the biomass yield was similar in both the cases. The strain accumulated 5.6 g L −1 of SA titer with a yield of 0.14 g g −1 ( Fig. 6 ). Acetic acid was obtained as the major product at higher quantities (8.3 g L −1 ) than the desired product SA. Further work to divert produced acetic acid towards SA is underway.
It is important to discuss two studies in recent times related to cost analysis for SA production from SCB. The sugar yield during pre-treatment has a significant impact on the commercial viability of LCB-based biorefineries. Nieder-Heitmann et al. (2020) investigated the profitability of different pre-treatment methods for SCB through simulation work using Aspen Plus. 124 They screened available pre-treatment technologies, and identified nine methods which were simulated and compared for the co-production of succinic acid and electricity in a SCB and trash biorefinery. The nine pre-treatment methods were as follows: dilute acid treatment (DAT) with enzymatic hydrolysis (EH); DAT without EH; NaOH with EH; organosolv with EH; ammonia fibre expansion with EH; steam explosion (STEX) with EH; STEX with SO 2 and EH; STEX with NaOH and EH; wet oxidation with EH. Except organosolv and wet oxidation, all other pre-treatment methods were found to be profitable. The most profitable was steam explosion with a SA yield, capital cost and internal rate of return (IRR) of 45.7 kg SA per 100 ton dry mass, $ 384.2 million and 28% respectively. The two challenges identified were proper mixing for efficient mass and heat transfer during pre-treatment and scale up of EH to the commercial level.
In another study, Klein et al. (2017) carried out simulation studies using Aspen Plus for the integration of SA production from SCB to optimized SCB biorefineries with the production of first generation (1G) ethanol and electricity. 125 SCB was pre-treated using dilute H 2 SO 4 and hemicellulosic hydrolysate (C5 liquor) obtained was fermented to SA by using wild type A. succinogenes after detoxification. The cellulignin fraction was utilized for electricity generation via CHP. The downstream step was designed and simulated based on literature data and resulted in a recovery of SA of up to 99%. The calculated cost of SA production was $2.32 per kg with sugarcane and capital cost being the major contributors. The calculated cost was similar to that of sugar-based SA production, $2.26 per kg SA from sucrose. Furthermore, the internal rate of return for the integrated SA biorefinery (15.8%) was lower than that of an ethanol distillery (17%). The 1G ethanol production is a mature and well-established technology; on the other hand, 2G SA production is in infancy and an emerging technology with lots of scope for improvement at the process as well as at the strain level.
Lactic acid (LA)
LA has also been receiving great attention as a feedstock for the manufacture of polylactic acid (PLA), a biodegradable polymer used as a raw material in packaging as well as fibres and foams. On an industrial scale, PLA production is considered a relatively immature technology as compared with petrochemical raw materials, mainly due to the high production cost of LA which is the raw material for PLA. The high costs of LA production are due to expensive sugar & nitrogen sources required for fermentation along with the downstream recovery and purification process. A major concern in LA fermentation is reducing the cost of the raw materials. This problem can be resolved through fermentative production of LA from low cost materials such as wastes from agricultural and agro-industrial residues. SCB can be the choice of raw material for LA production due to its low cost and well-established supply chain. However, most starchy and lignocellulose materials must be pre-treated by physicochemical and enzymatic methods as discussed in earlier sections. Also, LA from SCB will be economically attractive and competitive with LA production from pure sugars, if their productivities and yields are similar to those of pure sugar fermentation. It can only be accomplished when enzymatic liquefaction is performed at a high solid loading of pre-treated lignocellulosic biomass with uncompromised and concentrated sugar yields. Some of the factors that have a direct impact on enzyme hydrolysis are the type of pre-treatment 133,134 origin of the cellulases complex and accessory enzymes associated with it, pH, water availability and substrate feeding strategies. 135,136
LA production from SCB reported in the literature is summarised in Table 8 . Patel et al. (2005) used Bacillus sp. 36D1 (isolate) for LA production. Batch SSF of 20 g L −1 Solka Floc (commercial cellulose powder) with 10 FPU of Spezyme CE per g cellulose was carried out for 96 h by varying pH (4.5 to 7 at 50 °C) and temperature (30 to 60 °C at pH 5). 137 The volumetric productivity of LA was optimal between fermentation pH values of 4.5 and 5.5 at 50 °C. At a constant pH of 5, s LA titer of 18.3 g L −1 with s maximal LA volumetric productivity of 0.17 g L −1 h −1 was observed at 55 °C with L(+) isomer optical purity more than 95%. Furthermore, they also investigated the co-fermentation of cellulose-derived glucose and sugarcane bagasse hemicellulose derived xylose simultaneously (SSCF). In a batch SSCF of 40% acid pre-treated hemicellulose hydrolysate (over-limed) and 20 g L −1 Solka Floc cellulose, Bacillus sp. 36D1 produced about 35 g L −1 LA in about 144 h with 15 FPU of Spezyme CE per g cellulose.
To improve the LA titer and productivity, Adsul et al. (2007) have used steam and alkali pre-treated SCB at a higher solid loading and Lactobacillus delbrueckii mutant Uc-3. 138 Batch SSF of 80 g L −1 pre-treated SCB was conducted using a cellulase preparation (10 FPU per g of pre-treated SCB) derived from a mutant strain of Penicillium janthinellum . A LA titer of 67 g L −1 was obtained in 96 h from steam-alkali pre-treated SCB. In another study, Van der Pol et al. (2016) employed multiple strategies for the optimization of batch SSF of SCB obtained after acid pre-treatment and steam explosion. 139 They have investigated whether furfural addition (one of the inhibitors generated during pre-treatment) to precultures of Bacillus coagulans had a beneficial effect on the LA fermentation of pre-treated and enzyme hydrolysed SCB. The preculture was cultivated in PYPD medium with 1 g L −1 furfural. The pre-treated SCB solid fraction was hydrolysed with either a liquid fraction obtained from pre-treatment of SCB or demineralized water. Pre-treated SCB was hydrolysed with the enzyme cocktail GC220 and fermented by using B. coagulans DSM 2315 at pH 5.8 and 50 °C. For batch SSF of SCB with the liquid fraction from pre-treatment of SCB, a LA titer of 74.6 g L −1 and a productivity of 0.92 g L −1 h −1 were obtained for preculture in the PYPD medium with furfural as compared to a LA titer of 64.1 g L −1 and a productivity of 0.78 g L −1 h −1 for preculture in the PYPD medium. It was found that pre-cultivation in the presence of furfural was beneficial for LA fermentation. For batch SSF of pre-treated SCB, solid in water and preculture in the PYPD medium with furfural, the LA productivity increased to 1.14 g L −1 h −1 with 70.4 g L −1 of LA. The better productivity in the latter case was due to reduction in the lag phase from 40 h to 32 h.
Increasing the sugar concentration in hydrolysates can improve the productivity and yield of fermentation products. To achieve higher sugar concentrations in SCB hydrolysate, evaporation after hydrolysis or pre-treatment has been exploited as a strategy. Peng et al. (2014) concentrated enzyme hydrolysate of acid-alkali treated SCB. In their fed-batch fermentation experiments, an L(+) LA titer of 185 g L −1 with a productivity of 1.93 g L −1 h −1 was achieved with Bacillus sp. P38. In another study, de Oliveira et al. (2019) concentrated the hemicellulosic hydrolysate after acid pre-treatment of SCB five times. 131 For B. coagulans fermentation, an L(+) LA titer of 55.9 g L −1 with a yield of 0.87 g g −1 and a productivity of 1.7 g L −1 h −1 was obtained.
A range of bacterial strains such as Lactobacillus delbreuckii , Lactobacillus pentosus , Bacillus sp. and B. coagulans have been reported for LA fermentation ( Table 8 ). The optimal growth conditions for these bacteria are spread over the temperature range of 35–50 °C, but around neutral pH. The enzymatic hydrolysis conditions required to produce sugar rich hydrolysates however require radically different conditions, namely, temperature in the range of 45–55 °C and pH in the range of 4.5–5.5. Therefore, SSF as an approach for LA production from SCB would not be a feasible option as it compromises the operating parameters such as temperature and pH for either the hydrolysis stage or the fermentation stage. Hence, SHF can be a preferred approach over SSF especially for LA fermentation.
Along these lines, two strategies were evaluated for LA production in our consortium, 140 wherein pre-treatment of 12.5% SCB loading, Cellic CTec2 enzyme and thermophilic Bacillus coagulans NCIM 5648 were used. In first strategy, when Cellic CTec2 was dosed at 30 FPU g −1 of pre-treated SCB, it hydrolysed 75.8% cellulose and 88.6% xylan in 24 h. However, when the enzyme loading was changed to 25 mg protein per g glucan in the second case, it hydrolysed 72.3% and 68% cellulose and xylan respectively. The valorisation of glucose rich filtrates obtained from strategies 1 and 2 using two different media resulted in 50.4 g L −1 and 51.2 g L −1 of LA production from 54.7 to 62.7 g L −1 of glucose respectively. Despite opting for two alternative strategies during high solid SHF, an optically pure LA production of around 50 g L −1 was achieved within a short duration of 45–54 h from SCB hydrolysate.
In continuation to the above work in our consortium, a comparative study of four pre-treatments – alkali, acid–alkali, alkali–acid and HC in the presence of alkali – were conducted for SCB and their impact was evaluated based on sugar released from pre-treated SCB after enzymatic hydrolysis and subsequent LA production in a separate fermenter (SHF) by B. coagulans NCIM 5648 ( ref. 141 manuscript under review). The material balance from 100 g of untreated SCB to LA for alkali (A), acid–alkali (B), alkali–acid (C) and HC with alkali (D) pre-treatment routes is shown in Fig. 7 . After pre-treatment of SCB, 59 g, 32.4 g, 31.2 g and 62 g of pre-treated SCB were obtained for cases (A) through to (D) respectively. 17.5% (w/v) pre-treated SCB loading was used for enzymatic hydrolysis using the Cellic CTec2 enzyme. The enzyme hydrolysed SCB medium was used for LA fermentation using B. coagulans . Unlike most of the Bacillus strains reported earlier such as B. coagulans GKN316 and B. coagulans NL01, 142 B. coagulans NCIM 5648 was unable to valorise xylose to LA.
For the alkali pre-treatment (A), the recovery of 35.58 g cellulose, 8.6 g hemicellulose, and 8.1 g lignin in 59 g alkali treated SCB was achieved from 100 g untreated SCB. For the enzyme hydrolysis, 17.5 g of alkali treated SCB (17.5% w/v) was used. This resulted in a glucose release of 84.3 g L −1 with a hydrolysis efficiency of 72%. The enzyme hydrolysate was used for lactic acid fermentation using B. coagulans NCIM 5648. An L(+) LA titer, productivity and yield of 68.7 g L −1 , 2.86 g L −1 h −1 and 0.92 g g −1 were achieved respectively. From this route, 28.43 g of glucose was released during hydrolysis and 26.16 g of L(+) LA was produced. For the sequential alkali–acid pre-treatment (C), 23.4 g cellulose recovery in 31.2 g of alkali–acid treated SCB was obtained from 100 g untreated SCB. As mentioned earlier in (A), 17.5 g of alkali-acid treated SCB was used for enzyme hydrolysis. The highest sugar release of 89.3 g L −1 was achieved for the alkali–acid pre-treated SCB. After fermentation of enzyme hydrolysate, an L(+) LA titer, productivity and yield of 71.8 g L −1 , 2.99 g L −1 h −1 and 0.90 g g −1 were achieved respectively. This corresponded to 14.33 g L(+) LA. With cavitation in the presence of alkali (D), an L(+) LA titer of 62.5 g L −1 , productivity of 2.60 g L −1 h −1 and yield of 0.92 g g −1 were achieved. While (D) was similar to (A), sequential acid-alkali pre-treatment (B) was similar to (C). Considering the overall approach ( Fig. 7 and Table 8 ), alkali pre-treatment was found to be the best method for an enhanced L(+) LA yield. The product yield achieved was over 80% higher in comparison to the sequential pre-treatment. The material balance of multiple routes clearly shows that the substantial loss of the materials during any pre-treatment step may result in a lower economic efficiency of the overall process.
Summary and path forward
SCB, the predominant solid waste generated during sugarcane processing is a complex lignocellulosic biomass containing valuable components in cellulose and hemicellulose (interlinked to lignin) which requires significant pre-treatment. The pre-treatment of SCB is broadly grouped into two classes: first is for opening up the lignocellulosic matrix and enhancing the bioavailability of SCB. This is mainly used for recovering biogas–biomethane from SCB. HC is the most economical and recommended option for this class of pre-treatment. 41 The second class of pre-treatment is focussed on enhancing the enzymatic saccharification yield from SCB (C5 and C6 sugars). Mild acid hydrolysis in the presence of steam is the recommended option when C5 rich hydrolysate is the desired entity. 57,58 Enzymatic hydrolysis of the obtained cellulignin or subjecting it to alkali hydrolysis prior to enzymatic hydrolysis will yield a cellulose rich residue.
In an integrated biorefinery approach, C6 sugar (glucose) from SCB can be utilized for LA or ethanol production and C5 sugar (xylose) in hydrolysate may be utilized for SA or xylitol production or biogas production using anaerobic digestion. An integrated approach for the co-production of ethanol (from C6) and xylitol (from C5) from SCB is a promising starting point; 94 however further investigative efforts are required to progress further for realising these approaches on an industrial scale.
It is necessary to make use of microorganism(s), which can metabolize C6 as well as C5 sugars to maximize the bioconversion of lignocellulosic sugars. 87 Unlike glucose, xylose valorisation through a biochemical route is largely ignored, as most of the industrial microbes lack an efficient metabolic pathway for their assimilation. Furthermore, those microbial strains which can assimilate xylose suffer from a major setback due to preference for glucose as a carbon substrate over xylose. This suppresses xylose utilization during co-fermentation due to carbon catabolite repression (CCR). 97,149 Hence, more attention needs to be paid to the rewiring of metabolic networks of microbial strains to metabolize multiple carbon sources, especially glucose and xylose, from the feedstock which will be essential for de-risking the commercial viability of the bioprocesses. 150,151 In the last two decades, efforts on xylose valorisation have intensified. 152 To improve the efficacy of xylose-based fermentation, efficient xylose-utilizing as well as non-xylose/inefficient xylose-utilizing strains have been engineered to expand the available substrate range, enable rapid assimilation of xylose and eliminate CCR for manufacturing fuels and chemicals from LCB-based feedstock. 123,153,154 Despite advances in metabolic engineering and synthetic biology tools, xylose is still an inferior carbon source in comparison to glucose and more efforts are required to design efficient microbial cell factories for xylose-based production. Besides, the development of robust strains with a high tolerance level against inhibitors released during pre-treatment and end products can result in a high product yield which will eventually benefit the overall process economics. 151,152
Hydrolysis and fermentation processes need to be intensified for enhancing the productivity of SCB based biorefineries. The optimal conditions for hydrolysis and fermentation may be different and will vary for the enzyme complex used for hydrolysis and microbes used for fermentative production of desired products. Therefore, to achieve better combined productivity (hydrolysis & fermentation), selection of SHF vs. SSF is critical. For a specific case of LA production, SHF is recommended over SSF for better productivity. 140,141
The current state-of-the-art processes for transforming SCB into the value added products discussed here are still not yet economically feasible. 92–94,96,124,125 Major drivers for the deployment of biorefineries are sustainable & renewable energy supply, inclusive economic growth to save foreign exchange, less dependency on imported crude petroleum, low carbon footprint and green environment. Despite having high potential, the establishment of a biorefinery faces the following challenges. Firstly, round the year availability of lignocellulosic feedstock at a low price is a major concern for biorefineries. It is crucial to establish a proper mechanism for collection, transportation and handling of biomass feedstock. Secondly, 2G biorefinery investments are capital-intensive, involve large risks, and take a long time to become market ready. Finally, as discussed previously, pre-treatment and enzymatic hydrolysis can account for up to 40% of the total operational costs. Regarding the sugar industry in India, as far as the feedstock and supply-chain are concerned, the existing sugar mills have a well-established supply chain for sugarcane and hence readily available SCB on site. A typical sugar plant consists of a sugar unit, an ethanol production unit, a cogeneration plant (steam and power) and an anaerobic digestion unit (when a distillery is attached). These units facilitate the use of surplus steam, electricity and water on site. Typical equipment and process machinery required for expanding the plant are not different to those required for setting up biorefineries. They include fermenters, distillation columns, the evaporation plant, anaerobic digesters, etc. which can be used to setup biorefinery facilities. Saccharification modules required for the production of C5 or C6 sugars from polysaccharides may be the only new addition that is currently alien to sugar industries. Such a project can be a ‘bolt-on’ project which will be much cheaper than a Greenfield project. We believe that an AD centric biorefinery may overcome some of the limitations of the state-of-the-art and make the valorisation techno-economically viable. AD is an accepted technology in the sugar industry as it is used to operate digesters for processing spent wash from associated distilleries. AD is a robust technology and can handle fluctuations in feed quality and quantity. It hosts a consortium of microbes working in synergy and therefore can handle a wider range of pH and temperature. The capex requirements of AD are also one of the lowest among the competing technology platforms. The disadvantages of AD are the underlying transformation processes that are usually slow as they require large residence time, leading to larger digester volumes. It also offers limited control over substrate fraction selectivity. HC based pre-treatment can be used to intensify AD operation. It has been shown that HC based pre-treatment improves the rate as well as yield of biogas generation. One of the promising paths forward that we envisage is the use of AD not only as a bioprocess step for energy recovery but also as ‘pre-treatment’. In addition to recovering energy in the form of biogas–biomethane, it will also open up the lignocellulosic matrix of biomass (SCB). In the envisaged biorefinery, AD is used for only partially converting the organic carbon in the feed to biogas/biomethane. The digestate is processed further by separating the solid and liquid fractions, where the former will be subjected to pre-treatment for recovering C5 and C6 sugars and the latter can be sold as a fertiliser. C5 and C6 sugars produced from digestate can be used for generating high value products such as alcohols (ethanol and xylitol) and acids (LA and SA). It has to be ensured that the high value products produced from the ‘digestate’ should be of intended purity determined by the industry ( e.g. , 80–90% purity required for food grade lactic acid and > 90% for pharmaceutical grade 155 ) and free from contaminants. This proposed biorefinery is shown in Fig. 8 . The intended idea of using AD for partial conversion offers several advantages like smaller digesters due to a shorter residence time (required for partial conversion) and an inexpensive way to distort the lignocellulosic matrix for further bioprocessing. This has potential of substantially reducing the cost of conversion to sugars and thereby improving the overall economic viability. This approach may eliminate the need for harsh thermo-chemical treatments or expensive enzymatic treatment.
Further research, specifically on identifying optimal HC based pre-treatment suitable for partial conversion of organic carbon to biogas in AD, further cavitation based pre-treatment of digestate to make it amenable for extracting sugars and subsequent fermentation of sugars to desired products (alcohols and acids) are needed to realise the proposed biorefinery. We hope that this review and the proposed biorefinery will stimulate potential translation of ongoing research to practice in near future.
Declaration of interests
Conflicts of interest, acknowledgements.
- National Federation of Co-operative Sugar Factories Limited, Cooperative Sugar , National Federation of Cooperative Sugar Factories Ltd., New Delhi, 2019 Search PubMed .
- M. Meghana and Y. Shastri, Bioresour. Technol. , 2020, 303 , 122929 CrossRef CAS .
- S. Solomon, Sugar Tech , 2011, 13 , 255–265 CrossRef CAS .
- P. A. Ochoa George, J. J. C. Eras, A. S. Gutierrez, L. Hens and C. Vandecasteele, Waste Biomass Valorization , 2010, 1 , 407–413 CrossRef CAS .
- J. M. Hernández-Salas, M. S. Villa-Ramírez, J. S. Veloz-Rendón, K. N. Rivera-Hernández, R. A. González-César, M. A. Plascencia-Espinosa and S. R. Trejo-Estrada, Bioresour. Technol. , 2009, 100 , 1238–1245 CrossRef .
- A. Pandey, C. R. Soccol, P. Nigam and V. T. Soccol, Bioresour. Technol. , 2000, 74 , 69–80 CrossRef CAS .
- M. R. Trejo-Hernández, A. Ortiz, A. I. Okoh, D. Morales and R. Quintero, Chemosphere , 2007, 68 , 848–855 CrossRef .
- P. Purohit and S. Dhar, Promoting low carbon transport in India. Biofuel roadmap for India, United Nations Environment Programme-DTU Partnership, Climate and Sustainable Development , Technical University of Denmark, Magnum Custom Publishing, New Delhi, India, 2015, ISBN: 978-87-93130-66-1 Search PubMed .
- N. K. Bhardwaj, D. Kaur, S. Chaudhry, M. Sharma and S. Arya, J. Cleaner Prod. , 2019, 217 , 225–233 CrossRef CAS .
- H. C. J. Franco, M. T. B. Pimenta, J. L. N. Carvalho, P. S. G. Magalhães, C. E. V. Rossell, O. A. Braunbeck, A. C. Vitti, O. T. Kölln and J. Rossi Neto, Sci. Agric. , 2013, 70 , 305–312 CrossRef .
- P. Singh, A. Suman, P. Tiwari, N. Arya, A. Gaur and A. K. Shrivastava, World J. Microbiol. Biotechnol. , 2008, 24 , 667–673 CrossRef CAS .
- P. C. Abhilash and N. Singh, Bioresour. Technol. , 2008, 99 , 8961–8966 CrossRef CAS .
- S. Ezhumalai and V. Thangavelu, Bioresources , 2010, 5 , 1879–1894 CAS .
- S. Ingle, P. Ashtavinayak, D. Amol and P. Sanjay, J. Environ. Chem. Eng. , 2017, 5 , 2861–2868 CrossRef .
- S. Velmurugan and N. Partha, Indian Chem. Eng. , 2006, 48 , 160–163 Search PubMed .
- K. Umamaheswaran and V. S. Batra, Fuel , 2008, 87 , 628–638 CrossRef CAS .
- Ministry of New and Renewable Energy – Government of India, Programme/Scheme Wise Physical Progress in 2020–21 & Cumulative up to June, 2020 , https://mnre.gov.in/the-ministry/physical-progress, accessed 04 Sep, 2020 Search PubMed .
- M. R. Islam, H. Haniu, M. N. Islam and M. S. Uddin, J. Therm. Sci. Technol. , 2010, 5 , 11–23 CrossRef CAS .
- M. R. L. V. Leal, M. V. Galdos, F. V. Scarpare, J. E. A. Seabra, A. Walter and C. O. F. Oliveira, Biomass Bioenergy , 2013, 53 , 11–19 CrossRef .
- E. V. Gonçalves, F. L. Seixas, L. R. de Souza Scandiuzzi Santana, M. H. N. O. Scaliante and M. L. Gimenes, Can. J. Chem. Eng. , 2017, 95 , 1269–1279 CrossRef .
- D. Kumar, S. A. Soomro, H. U. Mahar, S. Aziz, I. N. Unar, A. R. Memon, A. A. Malik and S. Hussain, Technical Journal , 2017, 22 (2), 113–117 Search PubMed .
- A. Shukla and S. Y. Kumar, Int. J. Appl. Eng. Res. , 2017, 12 , 14739–14745 Search PubMed .
- A. K. Varma and P. Mondal, Ind. Crops Prod. , 2017, 95 , 704–717 CrossRef CAS .
- P. Ghorbannezhad, M. D. Firouzabadi, A. Ghasemian, P. J. de Wild and H. J. Heeres, J. Anal. Appl. Pyrolysis , 2018, 131 , 1–8 CrossRef CAS .
- S. Kanwal, N. Chaudhry, S. Munir and H. Sana, Waste Manag. , 2019, 88 , 280–290 CrossRef CAS .
- K. Manatura, Case Stud. Therm. Eng. , 2020, 19 , 100623 CrossRef .
- L. Venkateswar Rao, J. K. Goli, J. Gentela and S. Koti, Bioresour. Technol. , 2016, 213 , 299–310 CrossRef CAS .
- T. L. Bezerra and A. J. Ragauskas, Biofuels, Bioprod. Biorefin. , 2016, 10 , 634–647 CrossRef CAS .
- S. Farzad, M. A. Mandegari, M. Guo, K. F. Haigh, N. Shah and J. F. Görgens, Biotechnol. Biofuels , 2017, 10 , 87 CrossRef .
- B. Yang and C. E. Wyman, Biofuels, Bioprod. Biorefin. , 2008, 2 , 26–40 CrossRef CAS .
- A. S. A. da Silva, H. Inoue, T. Endo, S. Yano and E. P. S. Bon, Bioresour. Technol. , 2010, 101 , 7402–7409 CrossRef .
- S. Park, J. O. Baker, M. E. Himmel, P. A. Parilla and D. K. Johnson, Biotechnol. Biofuels , 2010, 3 , 10 CrossRef .
- Z. Miao, T. E. Grift, A. C. Hansen and K. C. Ting, Ind. Crops Prod. , 2011, 33 , 504–513 CrossRef CAS .
- R. d. R. O. d. Barros, R. d. S. Paredes, T. Endo, E. P. d. S. Bon and S.-H. Lee, Bioresour. Technol. , 2013, 136 , 288–294 CrossRef .
- B. Buaban, H. Inoue, S. Yano, S. Tanapongpipat, V. Ruanglek, V. Champreda, R. Pichyangkura, S. Rengpipat and L. Eurwilaichitr, J. Biosci. Bioeng. , 2010, 110 , 18–25 CrossRef CAS .
- Y. Sun and J. Cheng, Bioresour. Technol. , 2002, 83 , 1–11 CrossRef CAS .
- A. K. Kivaisi and S. Eliapenda, Renewable Energy , 1994, 5 , 791–795 CrossRef CAS .
- A. F. Leite, L. Janke, H. Harms, J. W. Zang, W. Fonseca-Zang, W. Stinner and M. Nikolausz, Energy Fuels , 2015, 29 , 4022–4029 CrossRef CAS .
- S. C. Rabelo, H. Carrere, R. Maciel Filho and A. C. Costa, Bioresour. Technol. , 2011, 102 , 7887–7895 CrossRef CAS .
- A. G. Costa, G. C. Pinheiro, F. G. C. Pinheiro, A. B. Dos Santos, S. T. Santaella and R. C. Leitão, Chem. Eng. J. , 2014, 248 , 363–372 CrossRef CAS .
- S. Nagarajan and V. V. Ranade, Ind. Eng. Chem. Res. , 2019, 58 , 15975–15988 CrossRef CAS .
- J. de Cassia Pereira, N. Paganini Marques, A. Rodrigues, T. Brito de Oliveira, M. Boscolo, R. da Silva, E. Gomes and D. A. Bocchini Martins, J. Appl. Microbiol. , 2015, 118 , 928–939 CrossRef CAS .
- D. Deswal, R. Gupta, P. Nandal and R. C. Kuhad, Carbohydr. Polym. , 2014, 99 , 264–269 CrossRef CAS .
- A. d. S. Machado and A. Ferraz, Bioresour. Technol. , 2017, 225 , 17–22 CrossRef CAS .
- C. Carrasco, H. M. Baudel, J. Sendelius, T. Modig, C. Roslander, M. Galbe, B. Hahn-Hägerdal, G. Zacchi and G. Lidén, Enzyme Microb. Technol. , 2010, 46 , 64–73 CrossRef CAS .
- G. J. M. Rocha, L. P. Andrade, C. Martín, G. T. Araujo, V. E. Mouchrek Filho and A. A. S. Curvelo, Ind. Crops Prod. , 2020, 147 , 112227 CrossRef CAS .
- A. M. Lali, A. A. Odaneth, S. H. Birhade, J. J. Victoria and S. C. Sawant, Process for production of soluble sugars from biomass, US Pat. US9862980B2, 2018.
- A. F. A. Carvalho, W. F. Marcondes, P. de Oliva Neto, G. M. Pastore, J. N. Saddler and V. Arantes, Bioresour. Technol. , 2018, 250 , 221–229 CrossRef CAS .
- P. V. Neves, A. P. Pitarelo and L. P. Ramos, Bioresour. Technol. , 2016, 208 , 184–194 CrossRef CAS .
- T. A. L. Silva, H. D. Z. Zamora, L. H. R. Varão, N. S. Prado, M. A. Baffi and D. Pasquini, Waste Biomass Valorization , 2018, 9 , 2191–2201 CrossRef CAS .
- P. Silva Ortiz and S. de Oliveira, Energy , 2014, 76 , 130–138 CrossRef .
- S. Nagarajan and V. V. Ranade, Bioresource Technology Reports , 2020, 100480, DOI: 10.1016/j.biteb.2020.100480 .
- K. Nakashima, Y. Ebi, N. Shibasaki-Kitakawa, H. Soyama and T. Yonemoto, Ind. Eng. Chem. Res. , 2016, 55 , 1866–1871 CrossRef CAS .
- V. V. Ranade, A. A. Kulkarni and V. M. Bhandari, Vortex diodes as effluent treatment devices, US Pat. US9422952B2, 2016.
- M. Langone, M. Soldano, C. Fabbri, F. Pirozzi and G. Andreottola, Appl. Biochem. Biotechnol. , 2018, 184 , 1200–1218 CrossRef CAS .
- M. Zieliński, P. Rusanowska, A. Krzywik, M. Dudek, A. Nowicka and M. Dębowski, Energies , 2019, 12 , 525 CrossRef .
- S. Pal, S. Joy, P. Kumbhar, K. D. Trimukhe, R. Gupta, R. C. Kuhad, A. J. Varma and S. Padmanabhan, Biomass Convers. Biorefin. , 2017, 7 , 179–189 CrossRef .
- S. Pal, W. J. Satyendra and R. P. Ravikumar, Preparation of hydrolysate of lignocellulosic materials, WIPO Patent WO2014203271A2, 2014.
- M. H. L. Silveira, A. K. Chandel, B. A. Vanelli, K. S. Sacilotto and E. B. Cardoso, Bioresource Technology Reports , 2018, 3 , 138–146 CrossRef .
- M. E. Vallejos, M. D. Zambon, M. C. Area and A. A. d. S. Curvelo, Ind. Crops Prod. , 2015, 65 , 349–353 CrossRef CAS .
- L. Mesa, E. González, C. Cara, M. González, E. Castro and S. I. Mussatto, Chem. Eng. J. , 2011, 168 , 1157–1162 CrossRef CAS .
- A. S. A. da Silva, S.-H. Lee, T. Endo and E. P. S. Bon, Bioresour. Technol. , 2011, 102 , 10505–10509 CrossRef .
- T. Mokomele, L. da Costa Sousa, V. Balan, E. Van Rensburg, B. E. Dale and J. F. Görgens, Biotechnol. Biofuels , 2018, 11 , 127 CrossRef .
- T. Benazzi, S. Calgaroto, V. Astolfi, C. Dalla Rosa, J. V. Oliveira and M. A. Mazutti, Enzyme Microb. Technol. , 2013, 52 , 247–250 CrossRef CAS .
- M. J. Madison, G. Coward-Kelly, C. Liang, M. N. Karim, M. Falls and M. T. Holtzapple, Biomass Bioenergy , 2017, 98 , 135–141 CrossRef CAS .
- O. Jafari and H. Zilouei, Bioresour. Technol. , 2016, 214 , 670–678 CrossRef CAS .
- K. Kapoor, N. Garg, R. K. Diwan, L. Varshney and A. K. Tyagi, Radiat. Phys. Chem. , 2017, 141 , 190–195 CrossRef CAS .
- T. Zhang and M.-J. Zhu, Bioresour. Technol. , 2016, 214 , 769–777 CrossRef CAS .
- M. Ambye-Jensen, R. Balzarotti, S. T. Thomsen, C. Fonseca and Z. Kádár, Biotechnol. Biofuels , 2018, 11 , 336 CrossRef CAS .
- P. J. Meynell, Methane: Planning a Digester , Schocken Books, New York, 1976 Search PubMed .
- S. Mittal, E. O. Ahlgren and P. R. Shukla, Energy Policy , 2018, 112 , 361–370 CrossRef CAS .
- Ministry of Petroleum Natural Gas Government of India, Energizing and Empowering India - Annual report 2018-19 , 2019, http://petroleum.nic.in/sites/default/files/AR_2018-19.pdf Search PubMed .
- Indian Oil Corporation Limited, Bharat Petroleum Corporation Limited and Hindustan Petroleum Corporation Limited, Expression of Interest from entrepreneurs/sole proprietorships/partnerships/limited liability partnerships/companies/cooperative societies/technology providers for production and supply of compressed biogas (CBG) under, SATAT , 2018, https://www.bharatpetroleum.com/pdf/EOICBGFINAL-46978-4fd490.pdf Search PubMed .
- H. Katuwal and A. K. Bohara, Renewable Sustainable Energy Rev. , 2009, 13 , 2668–2674 CrossRef .
- L. Janke, A. Leite, M. Nikolausz, T. Schmidt, J. Liebetrau, M. Nelles and W. Stinner, Int. J. Mol. Sci. , 2015, 16 , 20685 CrossRef CAS .
- S. Sajad Hashemi, K. Karimi and A. Majid Karimi, Fuel , 2019, 248 , 196–204 CrossRef CAS .
- A. M. Mustafa, H. Li, A. A. Radwan, K. Sheng and X. Chen, Bioresour. Technol. , 2018, 259 , 54–60 CrossRef CAS .
- M. Badshah, D. M. Lam, J. Liu and B. Mattiasson, Bioresour. Technol. , 2012, 114 , 262–269 CrossRef CAS .
- F. R. Ribeiro, F. Passos, L. V. A. Gurgel, B. E. L. Baêta and S. F. de Aquino, Sci. Total Environ. , 2017, 584–585 , 1108–1113 CrossRef CAS .
- L. Janke, A. F. Leite, M. Nikolausz, C. M. Radetski, M. Nelles and W. Stinner, J. Waste Manag. , 2016, 48 , 199–208 CrossRef CAS .
- M. Hassan, W. Ding, M. Umar and G. Rasool, Bioresour. Technol. , 2017, 230 , 24–32 CrossRef CAS .
- N. Aramrueang, J. Rapport and R. Zhang, Biosyst. Eng. , 2016, 147 , 174–182 CrossRef .
- P. V. Rao, S. S. Baral, R. Dey and S. Mutnuri, Renewable Sustainable Energy Rev. , 2010, 14 , 2086–2094 CrossRef CAS .
- C. Sawatdeenarunat, K. C. Surendra, D. Takara, H. Oechsner and S. K. Khanal, Bioresour. Technol. , 2015, 178 , 178–186 CrossRef CAS .
- A. F. Hernández-Pérez, I. A. L. Costa, D. D. V. Silva, K. J. Dussán, T. R. Villela, E. V. Canettieri, J. A. Carvalho, T. G. Soares Neto and M. G. A. Felipe, Bioresour. Technol. , 2016, 200 , 1085–1088 CrossRef .
- A. W. Bhutto, K. Harijan, K. Qureshi, A. A. Bazmi and A. Bahadori, J. Cleaner Prod. , 2015, 95 , 184–193 CrossRef CAS .
- L. Canilha, A. K. Chandel, T. S. S. Milessi, F. A. F. Antunes, W. L. C. Freitas, M. G. A. Felipe and S. S. da Silva, J. Biomed. Biotechnol. , 2012, 2012 Search PubMed .
- J. T. Cunha, P. O. Soares, A. Romaní, J. M. Thevelein and L. Domingues, Biotechnol. Biofuels , 2019, 12 , 20 CrossRef .
- R. E. Hector, J. A. Mertens, M. J. Bowman, N. N. Nichols, M. A. Cotta and S. R. Hughes, Yeast , 2011, 28 , 645–660 CrossRef CAS .
- A. de Araujo Guilherme, P. V. F. Dantas, C. E. d. A. Padilha, E. S. dos Santos and G. R. de Macedo, J. Environ. Manage. , 2019, 234 , 44–51 CrossRef .
- Y. Liu, J. X. Xu, Y. Zhang, M. He, C. Liang, Z. Yuan and J. Xie, BioResources , 2016, 11 , 2548–2556 CAS .
- O. Rosales-Calderon and V. Arantes, Biotechnol. Biofuels , 2019, 12 , 240 CrossRef .
- S. Vieira, M. V. Barros, A. C. N. Sydney, C. M. Piekarski, A. C. de Francisco, L. P. d. S. Vandenberghe and E. B. Sydney, Bioresour. Technol. , 2020, 299 , 122635 CrossRef CAS .
- A. F. Hernández-Pérez, P. V. de Arruda, L. Sene, S. S. da Silva, A. Kumar Chandel and M. d. G. de Almeida Felipe, Crit. Rev. Biotechnol. , 2019, 39 , 924–943 CrossRef .
- D. Dasgupta, D. Ghosh, S. Bandhu and D. K. Adhikari, Microbiol. Res. , 2017, 200 , 64–72 CrossRef CAS .
- Y. Xu, P. Chi, M. Bilal and H. Cheng, Appl. Microbiol. Biotechnol. , 2019, 103 , 5143–5160 CrossRef CAS .
- A. A. Prabhu, D. J. Thomas, R. Ledesma-Amaro, G. A. Leeke, A. Medina, C. Verheecke-Vaessen, F. Coulon, D. Agrawal and V. Kumar, Microb. Cell Fact. , 2020, 19 , 121 CrossRef CAS .
- A. A. Prabhu, E. Bosakornranut, Y. Amraoui, D. Agarwal, F. Coulon, V. Vivekanand, V. K. Thakur and V. Kumar, Biotechnol. Biofuels , 2020 Search PubMed , 10.21203/rs.3.rs-45506/v1, accepted.
- P. Unrean and N. Ketsub, Ind. Crops Prod. , 2018, 123 , 238–246 CrossRef CAS .
- D. D. V. da Silva, P. V. de Arruda, F. M. C. F. Vicente, L. Sene, S. S. da Silva and M. das Graças de Almeida Felipe, Ann. Microbiol. , 2015, 65 , 687–694 CrossRef .
- J. F. Castañón-Rodríguez, J. M. Domínguez-González, B. Ortíz-Muñiz, B. Torrestiana-Sanchez, J. A. R. de León and M. G. Aguilar-Uscanga, Eng. Life Sci. , 2015, 15 , 96–107 CrossRef .
- A. D. Mountraki, K. R. Koutsospyros, B. B. Mlayah and A. C. Kokossis, Waste Biomass Valorization , 2017, 8 , 2283–2300 CrossRef CAS .
- K.-K. Cheng, X.-B. Zhao, J. Zeng, R.-C. Wu, Y.-Z. Xu, D.-H. Liu and J.-A. Zhang, Appl. Microbiol. Biotechnol. , 2012, 95 , 841–850 CrossRef CAS .
- M. Babaei, K. Rueksomtawin Kildegaard, A. Niaei, M. Hosseini, S. Ebrahimi, S. Sudarsan, I. Angelidaki and I. Borodina, Front. Bioeng. Biotechnol. , 2019, 7 , 361 CrossRef .
- J. Akhtar, A. Idris and R. A. Aziz, Appl. Microbiol. Biotechnol. , 2014, 98 , 987–1000 CrossRef CAS .
- E. Stylianou, C. Pateraki, D. Ladakis, M. Cruz-Fernández, M. Latorre-Sánchez, C. Coll and A. Koutinas, Biotechnol. Biofuels , 2020, 13 , 72 CrossRef CAS .
- E. R. Borges and N. Pereira, J. Ind. Microbiol. Biotechnol. , 2011, 38 , 1001–1011 CrossRef CAS .
- Y.-l. Xi, W.-y. Dai, R. Xu, J.-h. Zhang, K.-q. Chen, M. Jiang, P. Wei and P.-k. Ouyang, Bioprocess Biosyst. Eng. , 2013, 36 , 1779–1785 CrossRef CAS .
- R. C. L. B. Rodrigues, M. G. A. Felipe, I. C. Roberto and M. Vitolo, Bioprocess Biosyst. Eng. , 2003, 26 , 103–107 CrossRef CAS .
- W. Carvalho, J. C. Santos, L. Canilha, J. B. Almeida e Silva, M. G. A. Felipe, I. M. Mancilha and S. S. Silva, Process Biochem. , 2004, 39 , 2135–2141 CrossRef CAS .
- R. S. Rao, C. P. Jyothi, R. S. Prakasham, P. N. Sarma and L. V. Rao, Bioresour. Technol. , 2006, 97 , 1974–1978 CrossRef CAS .
- C.-F. Huang, Y.-F. Jiang, G.-L. Guo and W.-S. Hwang, Bioresour. Technol. , 2011, 102 , 3322–3329 CrossRef CAS .
- G. Prakash, A. J. Varma, A. Prabhune, Y. Shouche and M. Rao, Bioresour. Technol. , 2011, 102 , 3304–3308 CrossRef CAS .
- M. E. Vallejos, M. Chade, E. B. Mereles, D. I. Bengoechea, J. G. Brizuela, F. E. Felissia and M. C. Area, Ind. Crops Prod. , 2016, 91 , 161–169 CrossRef CAS .
- V. P. de Arruda, J. C. dos Santos, R. de Cássia Lacerda Brambilla Rodrigues, D. D. V. da Silva, C. K. Yamakawa, G. J. de Moraes Rocha, J. N. Júnior, J. G. da Cruz Pradella, C. E. Vaz Rossell and M. das Graças de Almeida Felipe, J. Ind. Eng. Chem. , 2017, 47 , 297–302 CrossRef .
- P. Chen, S. Tao and P. Zheng, Bioresour. Technol. , 2016, 211 , 406–413 CrossRef CAS .
- W. Dessie, F. Xin, W. Zhang, Y. Jiang, H. Wu, J. Ma and M. Jiang, Appl. Microbiol. Biotechnol. , 2018, 102 , 9893–9910 CrossRef CAS .
- M. Sauer, D. Porro, D. Mattanovich and P. Branduardi, Trends Biotechnol. , 2008, 26 , 100–108 CrossRef CAS .
- E. Cavallo, H. Charreau, P. Cerrutti and M. L. Foresti, FEMS Yeast Res. , 2017, 17 , fox084 CrossRef .
- H.-H. Liu, X.-J. Ji and H. Huang, Biotechnol. Adv. , 2015, 33 , 1522–1546 CrossRef CAS .
- M. Egermeier, H. Russmayer, M. Sauer and H. Marx, Front. Microbiol. , 2017, 8 , 49 Search PubMed .
- K. L. Ong, C. Li, X. Li, Y. Zhang, J. Xu and C. S. K. Lin, Biochem. Eng. J. , 2019, 148 , 108–115 CrossRef CAS .
- A. A. Prabhu, R. Ledesma-Amaro, C. S. K. Lin, F. Coulon, V. K. Thakur and V. Kumar, Biotechnol. Biofuels , 2020, 13 , 113 CrossRef CAS .
- M. Nieder-Heitmann, K. Haigh, J. Louw and J. F. Görgens, Biofuels, Bioprod. Biorefin. , 2020, 14 , 55–77 CrossRef CAS .
- B. C. Klein, J. F. L. Silva, T. L. Junqueira, S. C. Rabelo, P. V. Arruda, J. L. Ienczak, P. E. Mantelatto, J. G. C. Pradella, S. V. Junior and A. Bonomi, Biofuels, Bioprod. Biorefin. , 2017, 11 , 1051–1064 CrossRef CAS .
- J. J. Bozell and G. R. Petersen, Green Chem. , 2010, 12 , 539–554 RSC .
- R. A. Abd Alsaheb, A. Aladdin, N. Z. Othman, R. Abd Malek, O. M. Leng, R. Aziz and H. A. El Enshasy, J. Chem. Pharm. Res. , 2015, 7 , 729–735 CAS .
- C. Rodrigues, L. P. S. Vandenberghe, A. L. Woiciechowski, J. de Oliveira, L. A. J. Letti and C. R. Soccol, in Current Developments in Biotechnology and Bioengineering , ed. A. Pandey, S. Negi and C. R. Soccol, Elsevier, 2017, pp. 543–556, DOI: 10.1016/B978-0-444-63662-1.00024-5 .
- T. Ghaffar, M. Irshad, Z. Anwar, T. Aqil, Z. Zulifqar, A. Tariq, M. Kamran, N. Ehsan and S. Mehmood, J. Radiat. Res. Appl. Sci. , 2014, 7 , 222–229 CrossRef CAS .
- B. Oonkhanond, W. Jonglertjunya, N. Srimarut, P. Bunpachart, S. Tantinukul, N. Nasongkla and C. Sakdaronnarong, J. Environ. Chem. Eng. , 2017, 5 , 2533–2541 CrossRef CAS .
- A. R. de Oliveira, R. Schneider, C. E. Vaz Rossell, R. Maciel Filho and J. Venus, Bioresource Technology Reports , 2019, 6 , 26–31 CrossRef .
- R. P. John, K. M. Nampoothiri and A. Pandey, Appl. Microbiol. Biotechnol. , 2007, 74 , 524–534 CrossRef CAS .
- L. J. Jonsson and C. Martin, Bioresour. Technol. , 2016, 199 , 103–112 CrossRef .
- A. K. Kumar and S. Sharma, Bioresour. Bioprocess , 2017, 4 , 7 CrossRef .
- M. R. Mukasekuru, J. Hu, X. Zhao, F. F. Sun, K. Pascal, H. Ren and J. Zhang, ACS Sustainable Chem. Eng. , 2018, 6 , 12787–12796 CrossRef CAS .
- D. H. Kim, H. M. Park, Y. H. Jung, P. Sukyai and K. H. Kim, Bioresour. Technol. , 2019, 284 , 391–397 CrossRef CAS .
- M. A. Patel, M. S. Ou, L. O. Ingram and K. T. Shanmugam, Biotechnol. Prog. , 2005, 21 , 1453–1460 CrossRef CAS .
- M. G. Adsul, A. J. Varma and D. V. Gokhale, Green Chem. , 2007, 9 , 58–62 RSC .
- E. C. van der Pol, G. Eggink and R. A. Weusthuis, Biotechnol. Biofuels , 2016, 9 , 248 CrossRef .
- K. Nalawade, P. Baral, S. Patil, A. Pundir, A. K. Kurmi, K. Konde, S. Patil and D. Agrawal, Renewable Energy , 2020, 157 , 708–717 CrossRef CAS .
- K. Nalawade, P. Saikia, S. Behera, K. S. Konde and S. Patil, Biomass Convers. Biorefin. , 2020, under review Search PubMed .
- T. Jiang, H. Qiao, Z. Zheng, Q. Chu, X. Li, Q. Yong and J. Ouyang, PLoS One , 2016, 11 , e0149101 CrossRef .
- W. Jonglertjunya, N. Srimarut, N. Lukkanakul, C. Sakdaronnarong and N. Nasongkla, Adv. Mater. Res. , 2014, 931–932 , 1597–1601 Search PubMed .
- L. Peng, N. Xie, L. Guo, L. Wang, B. Yu and Y. Ma, PLoS One , 2014, 9 , e107143 CrossRef .
- P. Unrean, Ind. Crops Prod. , 2018, 111 , 660–666 CrossRef CAS .
- D. Wischral, J. M. Arias, L. F. Modesto, D. de França Passos and N. Pereira Jr, Biotechnol. Prog. , 2019, 35 , e2718 CrossRef .
- A. González-Leos, M. G. Bustos-Vázquez, G. C. Rodríguez-Castillejos, L. V. Rodríguez-Durán and A. Del Ángel-Del Ángel, Rev. Mex. Ing. Quim. , 2019, 19 , 377–386 Search PubMed .
- D. Schneider and A. Ragossnig, Waste Manage. Res. , 2014, 32 , 563–564 CrossRef .
- C. Sievert, L. M. Nieves, L. A. Panyon, T. Loeffler, C. Morris, R. A. Cartwright and X. Wang, Proc. Natl. Acad. Sci. U. S. A. , 2017, 114 , 7349–7354 CrossRef CAS .
- F. M. Gírio, F. Carvalheiro, L. C. Duarte and R. Bogel-Łukasik, in D-xylitol: Fermentative Production, Application and Commercialization , ed. S. S. da Silva and A. K. Chandel, Springer Berlin Heidelberg, Berlin, Heidelberg, 2012, pp. 3–37, DOI: 10.1007/978-3-642-31887-0_1 .
- V. Kumar, P. Binod, R. Sindhu, E. Gnansounou and V. Ahluwalia, Bioresour. Technol. , 2018, 269 , 443–451 CrossRef CAS .
- S. Kwak, J. H. Jo, E. J. Yun, Y. S. Jin and J. H. Seo, Biotechnol. Adv. , 2019, 37 , 271–283 CrossRef CAS .
- R. G. de Paula, A. C. C. Antonieto, L. F. C. Ribeiro, N. Srivastava, A. O'Donovan, P. K. Mishra, V. K. Gupta and R. N. Silva, Biotechnol. Adv. , 2019, 37 , 107347 CrossRef CAS .
- S. Kwak and Y. S. Jin, Microb. Cell Fact. , 2017, 16 , 82 CrossRef .
- A. Ahmad, F. Banat and H. Taher, Environ. Technol. Innov. , 2020, 20 , 101138 CrossRef .
Academia.edu no longer supports Internet Explorer.
To browse Academia.edu and the wider internet faster and more securely, please take a few seconds to upgrade your browser .
Enter the email address you signed up with and we'll email you a reset link.
- We're Hiring!
- Help Center
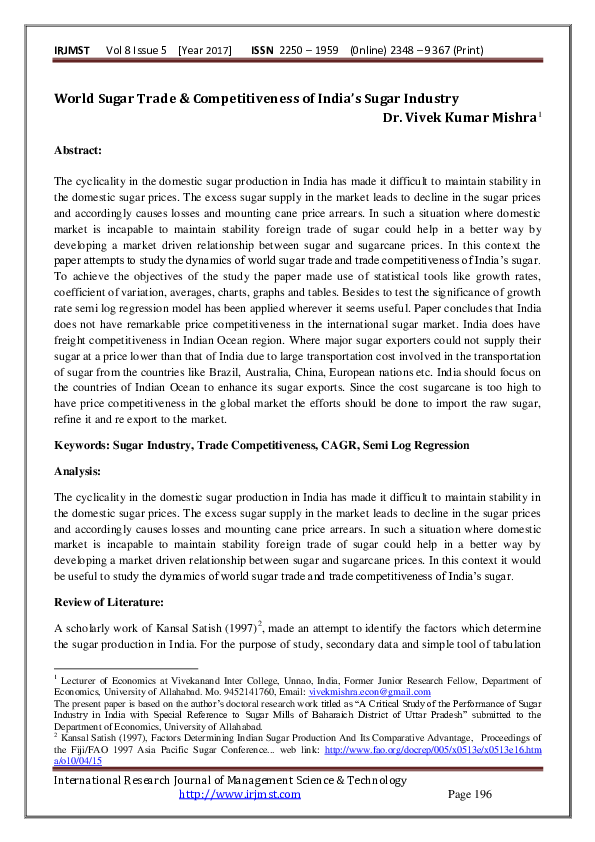
World Sugar Trade & Competitiveness of India’s Sugar Industry

The cyclicality in the domestic sugar production in India has made it difficult to maintain stability in the domestic sugar prices. The excess sugar supply in the market leads to decline in the sugar prices and accordingly causes losses and mounting cane price arrears. In such a situation where domestic market is incapable to maintain stability foreign trade of sugar could help in a better way by developing a market driven relationship between sugar and sugarcane prices. In this context the paper attempts to study the dynamics of world sugar trade and trade competitiveness of India's sugar. To achieve the objectives of the study the paper made use of statistical tools like growth rates, coefficient of variation, averages, charts, graphs and tables. Besides to test the significance of growth rate semi log regression model has been applied wherever it seems useful. Paper concludes that India does not have remarkable price competitiveness in the international sugar market. India does have freight competitiveness in Indian Ocean region. Where major sugar exporters could not supply their sugar at a price lower than that of India due to large transportation cost involved in the transportation of sugar from the countries like Brazil, Australia, China, European nations etc. India should focus on the countries of Indian Ocean to enhance its sugar exports. Since the cost sugarcane is too high to have price competitiveness in the global market the efforts should be done to import the raw sugar, refine it and re export to the market. The cyclicality in the domestic sugar production in India has made it difficult to maintain stability in the domestic sugar prices. The excess sugar supply in the market leads to decline in the sugar prices and accordingly causes losses and mounting cane price arrears. In such a situation where domestic market is incapable to maintain stability foreign trade of sugar could help in a better way by developing a market driven relationship between sugar and sugarcane prices. In this context it would be useful to study the dynamics of world sugar trade and trade competitiveness of India's sugar.
Related Papers
Interal Res journa Managt Sci Tech
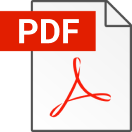
The present study represents the India's bilateral trade gap is increasing along with the country's overall trade gap with the rest of the world. It is important to examine to what extent is this bilateral trade imbalance contributing to the overall trade imbalance of India? How to sustain the present level of bilateral trade while at the same time narrowing the existing bilateral trade-gap is an important challenge for policy makers. India and China are the two fastest growing countries of the world, the possibility of an economic rapprochement among them to seize the synergies of their development is an important issue for research.
International Research Journal Commerce arts science
The term industrialization is widely accepted as an indicator of economic development because by the development of industries and with the creation of its linkage effects, the economy gets a prime moving force. This results a faster increase in economic development of an economy. The manufacturing sector which is an essential component of industrial sector is treated as the backbone of industrial development. If we trace out the development of industrial sector in India during the neo-liberalization period them it is clearly reflects that it makes an essential contribution in economics development. The contribution of industrial sector in GDP is approximately around 26.1 percent. India (with the exception of China) is currently the largest producer of textiles, chemical products, pharmaceuticals, basic metals, general machinery and equipment, and electrical machinery. In the coming year, the sector importance to the domestic and global economy is set to increase even further as a combination of supply-side advantages, policy initiatives, and private sector efforts set India on the path to a global manufacturing hub. But the growth rate of industrial sector is not consistent rather its rate has fluctuating or volatile over the time period. This happens due to there are several internal as well as external hindrances exist. This has resulted a pressure on trade approach and according to the commercial approach it is believed that if the performance of manufacturing sector is up to the mark than it will directly reflects the better performance in terms of export and import. Introduction Industrial sector is a key position in the Indian economy. The term industrialization is widely accepted as an indicator of economic development because by the development of industries and with the creation of its linkage effects, the economy gets a prime moving force. This results a faster increase in economic development of an economy. The industrial sector is an essential component of economic development. If we trace out the development of manufacturing sector in India during the neo-liberalization period them it is clearly reflects that it makes an essential contribution in industrial development. The contribution of manufacturing sector in GDP is approximately around 14 to 16 percent and its share in employment generation is about 12.0 per cent of the total India's labour force. India (with the exception of China) is currently the largest producer of textiles, chemical products, pharmaceuticals, basic metals, general machinery and equipment, and electrical machinery. The earlier perception about slow industrial growth during the last three years is at variance with the latest gross domestic product estimates, based on a new methodology and with 2011-12 as base year. The latter indicates an industrial recovery lead by manufacturing, electric and mining & quarrying However, in the current year; credit growth, corporate
Ever since the currency rates were reformed from theBretton woods systems where the currency of a country was tagged to the currency of another country or to gold, tothe present floating rate system, which takes a number of economic factors to determine the value of a currency, the Foreign Exchange Market has seen a growth in currency trade. With over $ 3Trillion trade on average every day, the FX market is one of the biggest financial markets. In this Paper titled ‘Facts and Figures of India’s falling Rupee’, the author looks into the various factors that determine the currency rate of a country and why the India Rupee has witnessed devaluation.
“Trade with other nations is essential for every economy in the world for meeting domestic needs as well as rest of the world. Global integration is the key characteristic of world economy nowadays. India, a developing economy, is contributing significantly in the world’s affairs and trade. Before 1991, more or less, this economy and its foreign dealings were not much opened but the wave of LPG of 1991 opened and changed the total scene of Indian economy drastically according to requirements. The extent along with direction and composition of foreign trade highly determines the situation of Indian economy. Against this backdrop, this attempt is to review and highlight some important facts and matters related to exports and imports of our nation. It covers two decade from 1991 to 2010 as reference time period. The study is mainly based on secondary data.”
The present study reveals that India and China are the two fastest growing countries of the world, the possibility of an economic rapprochement among them to seize the synergies of their development is an important issue for research. Both the countries have witnessed transitions in their economic policies during the last two-three decades and the irreversible nature of economic liberalization has enabled each nation to integrate with the world economy. While analyzing the existing patterns of their trade and sectorial complementariness for further economic engagement, the comparative macroeconomic performance of both economies may be examined in recent years need to be examined.
ABSRACT Foreign Direct Investment plays a major role in the advancement of technology, generating employment opportunities and promoting overall development of a country. Around 1990, India faced a huge BOP crisis due to which the policy makers felt the need to bring in the economic reforms in the name of Liberalization, Privatization and Globalization. This resulted in huge inflow of foreign capital into India. An enormous growth after the liberalization of the Indian economy has made FDI an important constituent of its growth and development strategy. The objective of this paper is to study the impact of FDI on the employment generation capacity in India and it also finds correlation between GDP and employment. This paper tries to make an analysis of India's growth pace in post reform period. It try to analyze that whether the employment generation in India after reforms may be attributed to this liberalization policy and to opening up of the economy to rest of the world or not.
FDI in India has a significant role in the economic growth and development of India. The trend in time series data of FDI inflow in India from 2000-01 to 2105-16 are analysed using parametric and non-parametric approaches. The time series were subjected to Man-Kendall test, Sen‘s slope test and regression analysis. The results showed significant increasing trend in total FDI inflow and FDI inflow in services, housing, telecommunication, computer and automobile sectors. The trend detected by Man-Kendall test and Sen‘s slope test are also reflected in the linear regression approach. The trend in total FDI inflow and automobile sector time series was found significant at 0.1 % level of significance, service sector at 1 % level of significance, computer and telecommunication at 5 % level of significance, where as housing sector at 10 % level of significance
Economist believes that Indian Agriculture sector achieved its growth upto remarkable level in last few decadess in the terms of Production, Output Growth etc. However there are various commodities in agriculture and allied sector which makes India Major producer in world market such as sugarcane, cotton, wheat, rice, spices, poultry farming, fisheries, livestock, milk, jute, pulses etc. The data of 12th five year plan shows that the target for the growth of agriculture and allied sector was 4.0 per cent annully and the actual achievement was 3.6 per cent annually in the GDP. Although data shows that agriculture and allied sector did not acheved its target which was 4.0 per cent but in case of production, it achieved a remarkable growth rate as compare to the ratio of population. World trade data shows that in a critical condition where other countries was facing slow down in agriculture sector India still has its positive growth in the export of its agriculture and allied sector commodieties. This paper is based on the secondary data which aims to analyse the pattern of growth of agriculuture and allied sector in India. Data have been collected from various government agencies, ministries, RBI etc.
RELATED PAPERS
Problemas Del Desarrollo
Raúl Ornelas
arXiv: General Relativity and Quantum Cosmology
Roberto Sussman Livovsky
Antar Bandyopadhyay
Daan Goense
Laboratorio Critico Rivista Di Francesistica
Massimo Blanco
marsella eka wardani
Journal of the American College of Cardiology
Miroslav Radulovic
Computers & Industrial Engineering
Surendra M. Gupta
Clinical Pediatrics and Research
Lilia Reyes
Croatica Chemica Acta
Marina Cindric
Developments in Strategic and Public Management
John Bryson
BMC Bioinformatics
Seval Ozkan
Observatorio de la Participacion IDPAC
Dynamic Systems and Applications
Mahmoud Osman
Journal of Advanced Research in Business and Management Studies
Noraza Mat Udin
Scientific Reports
Anna Bianchi
Travis Hannah, BSc PGD
Frontiers in Nutrition
Deepika Jeengar
Goldschmidt2021 abstracts
Anthony Gachetti
hjhjgfg freghrf
Darma Darma
Current Treatment Options in Oncology
Chemical Communications
Dr. Laksiri Weerasinghe
Nathaniel Burman
arXiv: General Mathematics
Subuhi Khan
RELATED TOPICS
- We're Hiring!
- Help Center
- Find new research papers in:
- Health Sciences
- Earth Sciences
- Cognitive Science
- Mathematics
- Computer Science
- Academia ©2024

- Personal Finance
- Today's Paper
- Partner Content
- Entertainment
- Social Viral
- Pro Kabaddi League
India likely to export almost 18 million tonnes of rice in 2024-25: USDA
Supplies increase year to year on record production at 527.6 million tons that more than offsets lower beginning stocks.
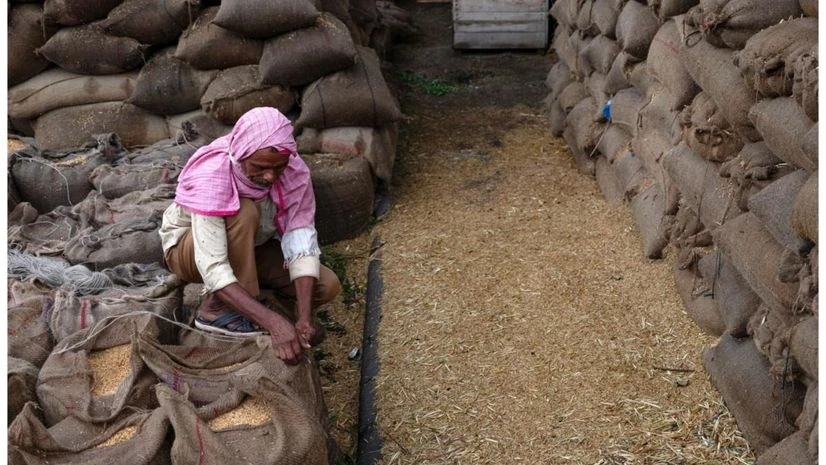)
Photo: Bloomberg
Listen to This Article
Slow purchases in some states drag rice procurement for central pool, centre keeping all options open to curb rice prices: food secretary, after curbs, non-basmati rice exports fell sharper than basmati rice, uttar pradesh govt transfers nearly rs 11,200 crore to paddy farmers, need for collective effort to improve dsr paddy cultivation: white paper, uttar pradesh govt plans to promote agri startups, introduce ai in farming, every rupee invested in agri research yields rs 13.85, says study, madhya pradesh farmers struggle to make the most of soaring wheat prices, govt lifts ban on onion exports; imposes min export price of $550 per tonne, centre imposes 40% duty on export of onions with effect from may 4.
Don't miss the most important news and views of the day. Get them on our Telegram channel
First Published: May 12 2024 | 11:38 PM IST
Explore News
- Suzlon Energy Share Price Adani Enterprises Share Price Adani Power Share Price IRFC Share Price Tata Motors Share Price Tata Steel Share Price Yes Bank Share Price Infosys Share Price SBI Share Price Tata Power Share Price
- Latest News Company News Market News India News Politics News Cricket News Personal Finance Technology News World News Industry News Education News Opinion Shows Economy News Lifestyle News Health News
- Today's Paper About Us T&C Privacy Policy Cookie Policy Disclaimer Investor Communication GST registration number List Compliance Contact Us Advertise with Us Sitemap Subscribe Careers BS Apps
- Budget 2024 Lok Sabha Election 2024 IPL 2024 Pro Kabaddi League IPL Points Table 2024

Evaluation of the Full-Frontal Crash Regulation for the M1 Category of Vehicles from an Indian Perspective
2024-01-2750.
- 1 What should be the appropriate test speed for the full-frontal test based on Indian accident data?
- 2 What is the suitable dummy configuration in terms of gender, seating position, and age to maximize occupant safety in full frontal accidents?
- 3 Is the proposed ATD’s anthropometry (weight and height) suitable, based on the people involved in full frontal cases in India?
- 4 What are occupant injury attributes in full-frontal accidents?
Advertisement
Advancement in Sugar Processing Technologies in India Emerging Innovations
- Feature Article
- Published: 10 November 2011
- Volume 13 , pages 378–393, ( 2011 )
Cite this article
- B. S. Gurumurthy 1
467 Accesses
2 Citations
Explore all metrics
Indian sugar industry has witnessed steady growth in terms of total production of sugar, total number of factories in operation, average plant size and to some extent in terms of duration and recovery. The growth has been steady and consistent when seen over a block of 4 to 5 years. The sugar industry in India has also progressively advanced in terms of innovation and technology development. This article has gone through a short discussion on every station so that one can understand as to how the sugar industry has systematically absorbed advanced technologies and innovations stage by stage with in the frame work of Government acts, rules and regulations. It has always responded well to the various assistance, incentives and subsidies for expansion, modernization, innovation and technology development. The industry has balanced well, the various issues that are always linked with but not under the control of the industry, like cane price and cane payment, sugar price and monthly releases, export/import of sugar and more importantly surplus and shortage situations.
This is a preview of subscription content, log in via an institution to check access.
Access this article
Price includes VAT (Russian Federation)
Instant access to the full article PDF.
Rent this article via DeepDyve
Institutional subscriptions
Similar content being viewed by others
Sugar Industry and Speciality Sugar Manufacturing
An overview of sugar sector in indonesia, development of sugar industry in asean countries, bibliography.
Anand, Mydur. 1982. Milling System 2001—A Concept Of The Future Mill 47 th Annual Convention, STAI.
Anand, M. 1987. Performance of Indian contipan, Proceedigs of Annual convention DSTA.
Gurumurthy, B.S., and M.S. Shivaswamy. 1975. Prospects of milling-cum-diffusion in India . Mumbai: Indian Sugar March.
Google Scholar
Gurumurthy, B. S., Srinivasan, S. 1986. Improved boiling techniques. In: Proceedings of 36th annual convention DSTA.
Gurumurthy, B. S., Vishweswara Rao, T. K. 2007. Optimising process steam demand-options. STAI All India Seminar on Heat Recovery.
Gurumurthy, B. S. 2009. Sugar manufacturing process technologies (with special reference to energy saving & sugar quality). In Successful implementation of steam economy measures, ed. L Bheemeshwara Rao. Sugar Journal, Annual convention of SISSTA.
Nikkam, Bhausaheb B., Nikam, Sachin B. 2008. Study of Field results of the unique two roller mill. 39th convention of SISSTA.
Download references
Aknowledgment
The aiuthor is delighted to profusely thank the president and members of IS 2011 for providing this opportunity.
Author information
Authors and affiliations.
Sugar Technologist, Bengaluru, Karnataka, India
B. S. Gurumurthy
You can also search for this author in PubMed Google Scholar
Corresponding author
Correspondence to B. S. Gurumurthy .
Rights and permissions
Reprints and permissions
About this article
Gurumurthy, B.S. Advancement in Sugar Processing Technologies in India Emerging Innovations. Sugar Tech 13 , 378–393 (2011). https://doi.org/10.1007/s12355-011-0106-0
Download citation
Received : 05 October 2011
Accepted : 12 October 2011
Published : 10 November 2011
Issue Date : December 2011
DOI : https://doi.org/10.1007/s12355-011-0106-0
Share this article
Anyone you share the following link with will be able to read this content:
Sorry, a shareable link is not currently available for this article.
Provided by the Springer Nature SharedIt content-sharing initiative
- Indian sugar industry
- Processing technology
- Advancements
- Find a journal
- Publish with us
- Track your research

IMAGES
VIDEO
COMMENTS
The South-Asian region including India is a major hub of sugar producing countries with ample presence in the global sugar scenario. India has a rich history of sugarcane and sugar production since time immemorial, and the industry has gradually evolved to find a place among the top sugar producing countries of the world. The innovative technological interventions for sugarcane improvement ...
Sugar industry is a seasonal industry operating for a maximum period of 5 - 6 months in a year. There are two methods through which sugar is produced in Indian sugar industries viz.1) Conventional double sulphitation process which produces direct consumption plantation white sugar, and 2) two-stage process in order to produce refined sugar. There are six major stages of manufacturing in ...
The global sugar market, at times, pivots around dynamics from within the Indian sugar industry. In 2022/23, India represents around 15.5% of global consumption. It is the world's largest consuming country, and its consumption total has increased by 10% since 2018/19. Meanwhile, Indian production has reached record output totals in 2021/22 ...
Sugarcane yield had negative growth during 7 out of 10 years of study. As a result, sugar industry had. shortage of sugarcane. Sugarcane yield (per hectare) in India was 59.10 tonnes during 2003 ...
The sugar industry is the most vital industry in India as well as in Uttar Pradesh. Its importance in the state can also be known from the fact that Uttar Pradesh is called the sugar bowl of India.
Abstract. Sugar industry is the second largest agro-based industry in India and contributes significantly to the socio-economic development of rural population. It supports 50 million farmers and ...
India is the largest consumer of sugar in the world. The sugar industry is amongst the most important agro-based industries in the country that impact livelihood of about 5 crore farmers and their family members and 5 lakh workers directly employed with the sugar mills. There are more than 700 installed sugar factories in the country with
The industry produces 370-400 million tons (MT) cane, 27-30 MT white sugar and 6-8 MT jaggery and khandsari every year. Besides, about 3.2 billion litres of alcohol and 4700 MW of power and many chemicals are also produced. The industry has the capability to export around 3500 MW of power to the national grid.
Sugar is the second largest agro-based industry in India and has a major influence on the country's water, food, and energy security. In this paper, we use a nexus approach to assess India's interconnected water-food-energy challenges, with a specific focus on the political economy of the sugar industry in Maharashtra, one of the country's largest sugar producing states.
S Pruthi 1995, in his research for Ph.D thesis, studied the history of sugar industry in India. The study focused on the history of sugar; sugar making in ancient and medieval India during the British period and after independence till 1992. He described various problems faced by the sugar industry in india.
Since 1930-31, the number of sugar mills in operation in India increased from 29 to 520, thereby leading to an increase in sugar production from 0.12 million to 32.82 million tonnes per year in 2018-19. 1 Today, the Indian sugar industry's annual output is worth approximately ₹800 billion (∼$12.5 billion).
with a record of more than 12.0% sugar recovery. in UP. The variety has occupied 2.2 million ha in. Uttar Pradesh that amounted to 82.21% of the. total sugarcane area in the state during 2019-20 ...
Present research paper is an attempt as to study of the progress of Sugar Industry in India, understand its problems and challenges in context on an going liberalization process. At present Indian Sugar Industry is facing a lot of problems. These are
The sugar industry in India may be divided ... in collaboration with Sugar Research Australia genomic selection, development of SNPs for ... This paper is a task to analyse the levels of ...
Mo. 9452141760, Email: [email protected] The present paper is based on the author's doctoral research work titled as ―A Critical Study of the Performance of Sugar Industry in India with Special Reference to Sugar Mills of Baharaich District of Uttar Pradesh‖ submitted to the Department of Economics, University of Allahabad. 2 ...
India is expected to produce 26.85MT sugar in 2019-2020 season as against 33.16 MT tonne produced in the previous year. The recent corona crisis (COVID-19) is adversely affecting the present and future activities of sugar industry by interfering with the national and international sugar and ethanol business.
India's rice exports will be a sizeable chunk of the global trade, but it will still be much lower than the record 22 million tonnes it exported in 2021-22, USDA said. On the world rice markets, it said the global rice outlook for 2024-25 is for rising supplies, trade, consumption, and ending stocks.
Page | 8 List of Figures Sl. No. Particulars Page No. 1 State share in production of and area under sugarcane in India during 2016-17 22 2 Demand and supply of sugar 23 3 FRP (₹ per quintal), A2+FL costs (₹ per quintal) and Recovery Rate (%) 25 4 Comparison between FRP and ex-mill prices (₹ per quintal) 26 5 International vs domestic prices of white sugar—October 2015 to June
Cogeneration i n Indian Sugar Industry: A Review. Suresh D. Mane. 1 Principal, Girijabai Sail Institute of Technology, Karwar, Karnataka, India 581345. Abstract. Cane industry is a large ...
Background: The Indian automobile industry, including the auto component industry, is a significant part of the country's economy and has experienced growth over the years.India is now the world's 3 rd largest passenger car market and the world's second-largest two-wheeler market. Along with the boon, the bane of road accident fatalities is also a reality that needs urgent attention, as ...
Indian sugar industry has witnessed steady growth in terms of total production of sugar, total number of factories in operation, average plant size and to some extent in terms of duration and recovery. The growth has been steady and consistent when seen over a block of 4 to 5 years. The sugar industry in India has also progressively advanced in terms of innovation and technology development ...
The research paper is based on the co-operative sugar factories in India. ... Since the sugar industry is one of India's oldest sectors and was developed throughout the Five Year Plans, several ...